
Chandrayaan-3: Oxygen, sulphur, iron, silicon; list of findings made on the Moon’s surface so far
Chandrayaan-3 rover confirms presence of sulphur on moon's surface; race against time for further experiments.

2. 4-meter diameter crater on Moon's surface: On 27 August, while plying on the Moon's surface, Chandrayaan-3 Rover faced an obstacle as it came across a 4-meter diameter crater. In an update from ISRO, it said the crater was positioned 3 meters ahead of its location. ISRO then decided to command the Rover to retrace the path and informed that the Rover was now safely heading on a new path.
Also Read: Ex-ISRO scientist credits PM Modi for Chandrayaan-3, says ‘previous govt didn't allocate funds’; Congress responds
3. Elements on the moon: On 30 August, the Laser-Induced Breakdown Spectroscope instrument onboard 'Pragyan' rover of Chandrayaan-3 ‘unambiguously confirmed’ the presence of sulphur in the lunar surface near south pole. Other elements like Aluminum (Al), Calcium (Ca), Iron (Fe), Chromium (Cr), Titanium (Ti), Manganese (Mn), Silicon (Si), and Oxygen (O) are also detected. The space agency further added that the search for Hydrogen (H) is underway.
Meanwhile, scientists have said that the rover is currently in a “race against time" with ISRO working to cover a maximum distance of the uncharted South pole through the six-wheeled vehicle. “We have only 14 days in total for this mission, which is equal to one day on the moon, so four days have been completed. The more experiments and research we can do in the remaining ten days will be important. We are in a race against time because in these 10 days, we have to do maximum work and all the ISRO scientists are working on it," Nilesh M Desai, Director, Space Applications Centre, told ANI on Sunday.
Earlier on Saturday, PM Modi had announced the decision to name the spot where Chandrayaan-3 Vikram lander made soft landing as ' Shiv Shakti Point ’ and the site where the Chandrayaan-2 lander crash-landed on the Moon's surface in 2019 would be known as "Tiranga Point". Also, August 23, the day the Chandrayaan-3 lander touched down on the lunar surface, would be celebrated as ‘National Space Day’, Modi had said.
(With inputs from agencies)
Catch all the Business News , Market News , Breaking News Events and Latest News Updates on Live Mint. Download The Mint News App to get Daily Market Updates.
Get Instant Loan up to ₹10 Lakh!
- Enter Mobile Number
Employment Type
Most active stocks, indian oil corporation share price, tata steel share price, reliance industries share price, tata motors share price, market snapshot.
- Top Gainers
- 52 Week High
City Union Bank share price
Federal bank share price, jubilant pharmova share price, uti asset management company share price, poly medicure share price, vodafone idea share price, chennai petroleum corporation share price, angel broking share price, fine organic industries share price, firstsource solutions share price, rainbow childrens medicare share price, gillette india share price, trending in market.
- Diwali 2024 Stock Picks
- Waaree Energies IPO
- Olectra Greentech stock
Recommended For You
Gold prices, popular in science, wait for it….
Log in to our website to save your bookmarks. It'll just take a moment.
Chandrayaan-3: A complete guide to India's third mission to the moon
A rover and lander will together explore the moon's surface.
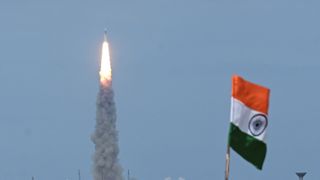
Chandrayaan-3 mission goals
Chandrayaan-3 science payloads, past chandrayaan missions, lessons learned from failed chandrayaan-2, additional resources.
Chandrayaan-3 is India's next moon mission.
The spacecraft launched to the moon on July 14, 2023, at 5:05 a.m. EDT (0905 GMT or 2:35 p.m. local time July 14) from the Satish Dhawan Space Center in Sriharikota, India atop the medium-lift Launch Vehicle Mark-III (LVM3) rocket.
Chandrayaan-3 successfully landed near the moon's south pole on Aug. 23, 2023, at 8:33 a.m. ET (1233 GMT or 6:03 p.m. India Standard Time).
The mission is managed by the Indian Space Research Organisation (ISRO). ISRO's roots go back to the beginning of space exploration, as a predecessor agency was set up in 1962 and its first rocket launch was in 1963. ISRO itself was established in 1969.
- India's Chandrayaan-3 moon lander shines in radar images from lunar orbit (photos)
- India Could Land on the Moon in 2020 with Chandrayaan 3
In June 2023, shortly before the scheduled Chandrayaan-3 launch, India also signed on to the NASA-led Artemis Accords aiming for peaceful human and robotic exploration of the moon. While the immediate benefits of the accords accrue to human spaceflight, according to the White House , the data from Chandrayaan-3 may be useful for future Artemis human landings too.
Related: Every mission to the moon
Chandrayaan-3 costs roughly $77 million USD, according to the Times of India .
The three main objectives of Chandrayaan-3 are to land safely on the surface, to demonstrate rover operations and to perform scientific experiments on site, according to the official website .
The mission called for a propulsion module to ferry the Chandrayaan-3' Vikram ("valor") lander and the solar-powered rover named Pragyan (Sanskrit for "wisdom") rover together to the south pole of the moon, according to NASA .
The module then entered lunar orbit and maneuvered into a roughly circular path about 60 miles (100 km) above the surface. Then the lander separated from the module and aimed for a soft landing on the surface, achieving this on Aug. 23, 2023.
The lander and rover will collect science on the surface for 14 Earth days (a single day on the moon), while the propulsion module will gaze at our planet for its own science experiment.
The spacecraft package (rover, lander and propulsion module) includes "advanced technologies" to meet the mission objectives, ISRO says. Examples include hazard detection and avoidance on the rover, a landing leg mechanism to aim for a soft touchdown, and altimeters and velocity instruments to estimate altitude and speed above the moon.
ISRO has performed several technology tests to simulate lunar conditions, the agency emphasized, focusing on matters such as soaking instruments in cold temperatures similar to the moon or doing a lander leg test on a simulated surface under different landing conditions.
Related: ISRO: The Indian Space Research Organization
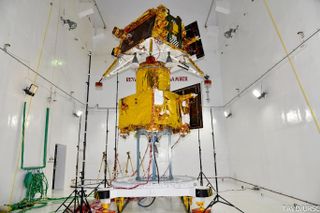
Science on the Chandrayaan-3 mission is split between the lander, the rover and the propulsion module payload.
"The lander is ... generally box-shaped, with four landing legs and four landing thrusters," NASA writes of the design . Its approximate 3,900-pound (1,752-kilogram) mass will include 57 pounds (26 kgs) for the rover.
The lander includes:
- Chandra's Surface Thermophysical Experiment (ChaSTE) to measure thermal conductivity and temperature on the surface;
- Instrument for Lunar Seismic Activity (ILSA) to detect moonquakes;
- Langmuir Probe to estimate the density and variation of plasma, or superheated gas, in the moon's environment;
- A Laser Retroreflector Array (from NASA) to measure distances using laser ranging..
The rover "is a rectangular chassis mounted on a six-wheel rocker-bogie wheel drive assembly," NASA added. The rover sends its communications to Earth through the lander. Rover instruments include:
- Alpha Particle X-ray Spectrometer (APXS) to look for elements in the lunar soil and rocks;
- Laser Induced Breakdown Spectroscope (LIBS) to examine the chemical and elemental composition of the lunar surface.
The propulsion module "is a box-like structure with one large solar panel mounted on one side and a large cylinder on top ... that acts as a mounting structure for the lander," NASA says. The propulsion module is more than 2.2 tons (2 tonnes in mass.)
The module's single experiment is the Spectro-polarimetry of Habitable Planet Earth (SHAPE) investigation that will assist with exoplanet searches. The experiment will "gather data on the polarization of light reflected by Earth so that researchers can look for other planets with similar signatures," according to Nature .
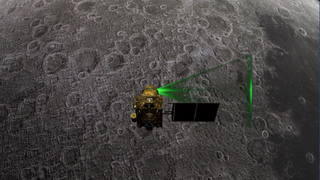
Chandrayaan-1 was India's first mission to the moon. It launched Oct. 22, 2008 from the Satish Dhawan Space Center in Sriharikota, India, aboard a Polar Satellite Launch Vehicle rocket. It achieved lunar orbit on Nov. 8. It released a Moon Impact Probe on Nov. 14 that deliberately crashed into the moon later that day.
Chandrayaan-1 is best known for finding evidence of water ice on the moon. NASA made the announcement on September 2009, based on data collected by the agency's Moon Mineralogy Mapper. The instrument found evidence of hydroxyl (a form of water, hydrogen and oxygen) in the moon's regolith or dust.
The Moon Impact Probe also found water's signature before impacting the surface, providing a separate set of data. More confirmations came from the Cassini spacecraft and the Deep Impact spacecraft's extended EPOXI mission.
Chandrayaan-2 was India's second mission to the moon. It launched from the Satish Dhawan Space Center in Sriharikota, India, aboard a Geosynchronous Satellite Launch Vehicle (GSLV) rocket on July 22, 2019. It made it to lunar orbit on Aug. 19, 2019.
On Sept. 6, Chandrayaan-2 released the Vikram moon lander , but mission officials lost contact with it as it was just 1.3 miles (2.1 km) above the surface. Although the lander was lost, the orbiter continues to work well. It carries eight different instruments and continues to send back high-definition imagery of the lunar surface.
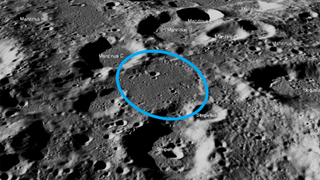
Chandrayaan-3 will build upon the "lessons learned" from the unsuccessful landing that took place during Chandrayaan-2, ISRO told the Business Standard .
"With optimized payload configurations, improved lander capabilities, and utilizing existing (spacecraft) resources, the mission is expected to address past challenges," the Business Standard wrote of ISRO's approach to Chandrayaan-3.
For example, Chandrayaan-3 will simplify its mission design to not include an orbiter. The predecessor mission, Chandrayaan-2, will therefore handle all communications to Earth from the propulsion module, the rover and the lander.
The propulsion module ferrying Chandraayan-3 to the moon will also only include a single science instrument, as opposed to Chandrayaan-2's orbiter which carried nine. This will simplify the amount of work the propulsion module performs, allowing engineers to focus on its crucial role in bringing the rover and lander to the moon.
The lander of Chandraayan-3 also includes key upgrades. ISRO stated it will have two "lander hazard detection and avoidance cameras" meant to help the lander avoid obstacles on the surface during the descent. Chandrayaan-2 only carried one such camera, and Chandrayaan-3's cameras aim to be more robust than the predecessor mission.
Read more about Chandraayan-3 on the official ISRO website . NASA has technical details about the mission as well.
Bibliography
The Business Standard. (2023, July 7). "Chandrayaan-3: What is it, and how does it improve on its predecessor?" https://www.business-standard.com/india-news/chandrayaan-3-what-is-it-and-how-does-it-improve-on-its-predecessor-123070700477_1.html
Padma, T.V. (2023, July 7). "India shoots for the moon with Chandrayaan-3 lunar lander." Nature . https://www.nature.com/articles/d41586-023-02217-0
The White House. (2023, June 22.) "Republic of India official state visit to the United States." https://www.whitehouse.gov/briefing-room/statements-releases/2023/06/22/fact-sheet-republic-of-india-official-state-visit-to-the-united-states/
Times of India. (2023, July 6). "Chandrayaan-3 launch on July 14; August 23-24 preferred landing dates." http://timesofindia.indiatimes.com/articleshow/101547465.cms
Times of India. (2020, Jan. 2.) "Chandrayaan-3 to cost Rs 615 crore, launch could stretch to 2021." https://timesofindia.indiatimes.com/india/chandrayaan-3-to-cost-rs-615-crore-launch-could-stretch-to-2021/articleshow/73055941.cms
Join our Space Forums to keep talking space on the latest missions, night sky and more! And if you have a news tip, correction or comment, let us know at: [email protected].
Get the Space.com Newsletter
Breaking space news, the latest updates on rocket launches, skywatching events and more!
Elizabeth Howell (she/her), Ph.D., is a staff writer in the spaceflight channel since 2022 covering diversity, education and gaming as well. She was contributing writer for Space.com for 10 years before joining full-time. Elizabeth's reporting includes multiple exclusives with the White House and Office of the Vice-President of the United States, an exclusive conversation with aspiring space tourist (and NSYNC bassist) Lance Bass, speaking several times with the International Space Station, witnessing five human spaceflight launches on two continents, flying parabolic, working inside a spacesuit, and participating in a simulated Mars mission. Her latest book, " Why Am I Taller ?", is co-written with astronaut Dave Williams. Elizabeth holds a Ph.D. and M.Sc. in Space Studies from the University of North Dakota, a Bachelor of Journalism from Canada's Carleton University and a Bachelor of History from Canada's Athabasca University. Elizabeth is also a post-secondary instructor in communications and science at several institutions since 2015; her experience includes developing and teaching an astronomy course at Canada's Algonquin College (with Indigenous content as well) to more than 1,000 students since 2020. Elizabeth first got interested in space after watching the movie Apollo 13 in 1996, and still wants to be an astronaut someday. Mastodon: https://qoto.org/@howellspace
North Korea launches intercontinental ballistic missile to space, reaches record altitude
Apollo 11 moonwalker Buzz Aldrin endorses Trump for president
Watch SpaceX Crew-9 astronauts move Crew Dragon spacecraft to new ISS parking spot on Nov. 3
- SpaceManTom YES! I can’t wait for the entire Live Feed pointing back at Earth. This will be Amazing to see. Reply
- View All 1 Comment
Most Popular
- 2 Space isn’t all about the 'race' – rival superpowers must work together for a better future
- 3 Every upcoming Star Wars game officially announced
- 4 This Week In Space podcast: Episode 135 — The Spacer Pipeline
- 5 NASA astronaut captures city lights streaking below ISS in stunning new photos
Thank you for visiting nature.com. You are using a browser version with limited support for CSS. To obtain the best experience, we recommend you use a more up to date browser (or turn off compatibility mode in Internet Explorer). In the meantime, to ensure continued support, we are displaying the site without styles and JavaScript.
- View all journals
- Explore content
- About the journal
- Publish with us
- Sign up for alerts
- Published: 21 August 2024
Chandrayaan-3 APXS elemental abundance measurements at lunar high latitude
- Santosh V. Vadawale ORCID: orcid.org/0000-0002-2050-0913 1 ,
- N. P. S. Mithun ORCID: orcid.org/0000-0003-3431-6110 1 ,
- M. Shanmugam ORCID: orcid.org/0000-0002-5995-8681 1 ,
- Amit Basu Sarbadhikari ORCID: orcid.org/0000-0002-6935-1381 1 ,
- Rishitosh K. Sinha ORCID: orcid.org/0000-0002-4545-7069 1 ,
- Megha Bhatt ORCID: orcid.org/0000-0002-6237-1072 1 ,
- S. Vijayan ORCID: orcid.org/0000-0002-1028-8074 1 ,
- Neeraj Srivastava 1 ,
- Anil D. Shukla 1 , 2 ,
- S. V. S. Murty ORCID: orcid.org/0000-0002-9835-3415 1 ,
- Anil Bhardwaj ORCID: orcid.org/0000-0003-1693-453X 1 ,
- Y. B. Acharya 1 ,
- Arpit R. Patel ORCID: orcid.org/0000-0002-0929-1401 1 ,
- Hiteshkumar L. Adalaja ORCID: orcid.org/0000-0002-5272-6386 1 ,
- C. S. Vaishnava ORCID: orcid.org/0000-0002-8096-5683 1 ,
- B. S. Bharath Saiguhan ORCID: orcid.org/0000-0001-7580-364X 1 ,
- Nishant Singh ORCID: orcid.org/0000-0002-1975-0552 1 ,
- Sushil Kumar ORCID: orcid.org/0009-0004-2604-9635 1 ,
- Deepak Kumar Painkra ORCID: orcid.org/0009-0009-8350-491X 1 ,
- Yash Srivastava ORCID: orcid.org/0000-0003-3793-8951 1 ,
- Varsha M. Nair ORCID: orcid.org/0009-0000-6684-2671 1 ,
- Tinkal Ladiya ORCID: orcid.org/0000-0001-6022-8283 1 ,
- Shiv Kumar Goyal 1 ,
- Neeraj K. Tiwari 1 ,
- Shyama Narendranath ORCID: orcid.org/0000-0001-9199-4925 3 ,
- Netra S. Pillai 3 ,
- Arup Kumar Hait 4 ,
- Aaditya Patinge ORCID: orcid.org/0009-0008-7700-9069 4 ,
- Abhishek Kumar 3 ,
- Neeraj Satya 3 ,
- Vivek R. Subramanian 3 ,
- Sonal G. Navle 3 ,
- R. G. Venkatesh 3 ,
- Lalitha Abraham 3 ,
- K. Suresh ORCID: orcid.org/0000-0003-3994-1003 4 &
- Amitabh 4
Nature volume 633 , pages 327–331 ( 2024 ) Cite this article
7160 Accesses
1060 Altmetric
Metrics details
- Geochemistry
- Rings and moons
The elemental composition of the lunar surface provides insights into mechanisms of the formation and evolution of the Moon 1 , 2 . The chemical composition of lunar regolith have so far been precisely measured using the samples collected by the Apollo, Luna and Chang’e 5 missions, which are from equatorial to mid-latitude regions 3 , 4 ; lunar meteorites, whose location of origin on the Moon is unknown 5 , 6 ; and the in situ measurement from the Chang’e 3 and Chang’e 4 missions 7 , 8 , 9 , which are from the mid-latitude regions of the Moon. Here we report the first in situ measurements of the elemental abundances in the lunar southern high-latitude regions by the Alpha Particle X-ray Spectrometer (APXS) experiment 10 aboard the Pragyan rover of India’s Chandrayaan-3 mission. The 23 measurements in the vicinity of the Chandrayaan-3 landing site show that the local lunar terrain in this region is fairly uniform and primarily composed of ferroan anorthosite (FAN), a product of the lunar magma ocean (LMO) crystallization. However, observation of relatively higher magnesium abundance with respect to calcium in APXS measurements suggests the mixing of further mafic material. The compositional uniformity over a few tens of metres around the Chandrayaan-3 landing site provides an excellent ground truth for remote-sensing observations.
This is a preview of subscription content, access via your institution
Access options
Access Nature and 54 other Nature Portfolio journals
Get Nature+, our best-value online-access subscription
24,99 € / 30 days
cancel any time
Subscribe to this journal
Receive 51 print issues and online access
185,98 € per year
only 3,65 € per issue
Buy this article
- Purchase on SpringerLink
- Instant access to full article PDF
Prices may be subject to local taxes which are calculated during checkout
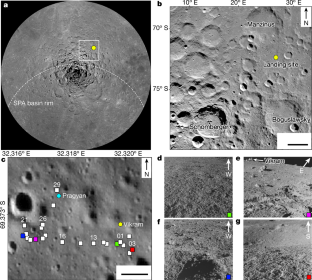
Similar content being viewed by others
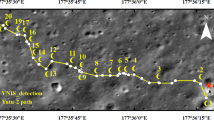
Mineralogical and chemical properties inversed from 21-lunar-day VNIS observations taken during the Chang’E-4 mission
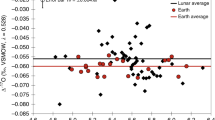
Distinct oxygen isotope compositions of the Earth and Moon
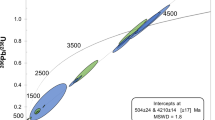
Lunar samples record an impact 4.2 billion years ago that may have formed the Serenitatis Basin
Data availability.
APXS raw data and calibrated spectral files, along with the necessary calibration data for abundance estimation, will be available publicly at the Chandrayaan-3 portal of the ISRO Science Data Archive at https://pradan.issdc.gov.in/ch3/ from 23 August 2024. Chandrayaan-2 Orbiter High Resolution Camera (OHRC) data are available at https://pradan.issdc.gov.in/ch2/ (file id ch2_ohr_ncp_20211023T002746282). Data from the LRO WAC, Chandrayaan-1 M 3 , Kaguya TC and Clementine UVVIS instruments used in this publication are publicly available in the Planetary Data System (PDS) archives. Source data for Fig. 2 and Fig. 3 are provided with this paper.
Code availability
Spectral analysis carried out in this work uses the Xspec spectral fitting package ( https://heasarc.gsfc.nasa.gov/xanadu/xspec/ ). Further analysis and plotting made use of the open-source Python packages numpy, scipy, matplotlib and pyrolite.
Shearer, C. K. Thermal and magmatic evolution of the Moon. Rev. Mineral. Geochem. 60 , 365–518 (2006).
Article CAS Google Scholar
Lucey, P. Understanding the lunar surface and space-Moon interactions. Rev. Mineral. Geochem. 60 , 83–219 (2006).
Vaniman, D., Dietrich, J., Taylor, G. J. & Heiken, G. in Lunar Sourcebook, A User’s Guide to the Moon (eds Heiken, G. H., Vaniman, D. T. & French, B. M.) 5–26 (Cambridge Univ. Press, 1991).
Qian, Y. et al. The regolith properties of the Chang’e-5 landing region and the ground drilling experiments using lunar regolith simulants. Icarus 337 , 113508 (2020).
Korotev, R. Lunar geochemistry as told by lunar meteorites. Geochemistry 65 , 297–346 (2005).
Joy, K. H. et al. Lunar meteorites. Rev. Mineral. Geochem. 89 , 509–562 (2023).
Article Google Scholar
Ip, W.-H., Yan, J., Li, C.-L. & Ouyang, Z.-Y. Preface: The Chang’e-3 lander and rover mission to the Moon. Res. Astron. Astrophys. 14 , 1511–1513 (2014).
Article ADS Google Scholar
Wu, W. et al. Lunar farside to be explored by Chang’e-4. Nat. Geosci. 12 , 222–223 (2019).
Article ADS CAS Google Scholar
Li, C. et al. Chang’E-4 initial spectroscopic identification of lunar far-side mantle-derived materials. Nature 569 , 378–382 (2019).
Article ADS CAS PubMed Google Scholar
Shanmugam, M. et al. Alpha particle X-ray spectrometer onboard Chandrayaan-2 rover. Curr. Sci. 118 , 53 (2020).
Meyer, H., Denevi, B., Robinson, M. & Boyd, A. The global distribution of lunar light plains from the lunar reconnaissance orbiter camera. J. Geophys. Res. Planets 125 , e2019JE006073 (2020).
Spudis, P. D., Reisse, R. A. & Gillis, J. J. Ancient multiring basins on the Moon revealed by Clementine laser altimetry. Science 266 , 1848–1851 (1994).
Speyerer, E. J., Robinson, M. S., Boyd, A., Wagner, R. V. & Henriksen, M. R. Exploration of the lunar south pole with LROC data products. Lunar Surface Science Workshop, LPI Contribution No. 2241, id.5132 (2020).
Sinha, R. K., Rani, A., Ruj, T. & Bhardwaj, A. Geologic investigation of lobate scarps in the vicinity of Chandrayaan-3 landing site in the southern high latitudes of the moon. Icarus 402 , 115636 (2023).
Durga Prasad, K. et al. Contextual characterization study of Chandrayaan-3 primary landing site. Mon. Not. R. Astron. Soc. 526 , L116–L123 (2023).
ADS Google Scholar
Mithun, N. P. S. et al. Ground calibration of Alpha Particle X-ray Spectrometer (APXS) on-board Chandrayaan-2 Pragyaan rover: an empirical approach. Planet. Space Sci. 187 , 104923 (2020).
Korotev, R. L. Some things we can infer about the Moon from the composition of the Apollo 16 regolith. Meteorit. Planet. Sci. 32 , 447–478 (1997).
Laul, J. C. & Schmitt, E. A. Chemical composition of Luna 20 rocks and soil and Apollo 16 soils. Geochim. Cosmochim. Acta 37 , 927–942 (1973).
Radhakrishna, V. et al. Chandrayaan-2 large area soft X-ray spectrometer. Curr. Sci. 118 , 219–225 (2020).
Pieters, C. et al. The Moon Mineralogy Mapper (M 3 ) on Chandrayaan-1. Curr. Sci. 96 , 500–505 (2009).
CAS Google Scholar
Bansal, B. M. et al. The chemical composition of soil from the Apollo 16 and Luna 20 sites. Earth Planet. Sci. Lett. 17 , 29–35 (1972).
Wood, J. A., Dickey, J. S. Jr, Marvin, U. B. & Powell, B. N. Lunar anorthosites and a geophysical model of the moon. Geochim. Cosmochim. Acta Suppl. 1 , 965 (1970).
ADS CAS Google Scholar
Warren, P. H. The magma ocean concept and lunar evolution. Annu. Rev. Earth Planet. Sci. 13 , 201–240 (1985).
Wood, J. A. in Origin of the Moon (eds Hartmann, W. K., Phillips, R. J. & Taylor, G. J.) 17–55 (Lunar and Planetary Institute, 1986).
Srivastava, Y., Basu Sarbadhikari, A., Day, J. M., Yamaguchi, A. & Takenouchi, A. A changing thermal regime revealed from shallow to deep basalt source melting in the Moon. Nat. Commun. 13 , 7594 (2022).
Article ADS CAS PubMed PubMed Central Google Scholar
Ohtake, M. et al. The global distribution of pure anorthosite on the Moon. Nature 461 , 236–240 (2009).
Donaldson Hanna, K. L. et al. Global assessment of pure crystalline plagioclase across the Moon and implications for the evolution of the primary crust. J. Geophys. Res. Planets 119 , 1516–1545 (2014).
Warren, P. H. Lunar anorthosites and the magma-ocean plagioclase-flotation hypotheses: importance of FeO enrichment in the parent magma. Am. Mineral. 75 , 46–58 (1990).
Warren, P. H. A concise compilation of petrologic information on possibly pristine nonmare Moon rocks. Am. Mineral. 78 , 360–376 (1993).
McCallum, I. S. A new view of the Moon in light of data from Clementine and Prospector missions. Earth Moon Planets 85 , 253–269 (2001).
Cross, W., Iddings, J. P., Pirsson, L. V. & Washington, H. S. A quantitative chemico-mineralogical classification and nomenclature of igneous rocks. J. Geol. 10 , 555–690 (1902).
Verma, S. P., Torres-Alvarado, I. S. & Velasco-Tapia, F. A revised CIPW norm. Swiss Bull. Mineral. Petrol. 83 , 197–216 (2003).
Warren, P. H. & Korotev, R. L. Ground truth constraints and remote sensing of lunar highland crust composition. Meteorit. Planet. Sci. 57 , 527–557 (2022).
Meyer, C. The lunar sample compendium. NASA https://curator.jsc.nasa.gov/Lunar/lsc/index.cfm (2012).
Lemelin, M., Lucey, P. G. & Camon, A. Compositional maps of the lunar polar regions derived from the Kaguya Spectral Profiler and the Lunar Orbiter Laser Altimeter data. Planet. Sci. J. 3 , 63 (2022).
Wieczorek, M. A. & Zuber, M. T. The composition and origin of the lunar crust: constraints from central peaks and crustal thickness modeling. Geophys. Res. Lett. 28 , 4023–4026 (2001).
Wieczorek, M. A. The constitution and structure of the lunar interior. Rev. Mineral. Geochem. 60 , 221–364 (2006).
Dygert, N., Lin, J.-F., Marshall, E. W., Kono, Y. & Gardner, J. E. A low viscosity lunar magma ocean forms a stratified anorthitic flotation crust with mafic poor and rich units. Geophys. Res. Lett. 44 , 11,282–11,291 (2017).
Tompkins, S. & Pieters, C. M. Mineralogy of the lunar crust: results from Clementine. Meteorit. Planet. Sci. 34 , 25–41 (1999).
Stuart-Alexander, D. E. Geologic map of the central far side of the Moon. U.S. Geological Survey, IMAP 1047 (1978).
Yamamoto, S. et al. Possible mantle origin of olivine around lunar impact basins detected by SELENE. Nat. Geosci. 3 , 533–536 (2010).
McGetchin, T. R., Settle, M. & Head, J. W. Radial thickness variation in impact crater ejecta: implications for lunar basin deposits. Earth Planet. Sci. Lett. 20 , 226–236 (1973).
Fassett, C. I., Head, J. W., Smith, D. E., Zuber, M. T. & Neumann, G. A. Thickness of proximal ejecta from the Orientale Basin from Lunar Orbiter Laser Altimeter (LOLA) data: implications for multi-ring basin formation. Geophys. Res. Lett. 38 , L17201 (2011).
Klima, R. L. et al. New insights into lunar petrology: distribution and composition of prominent low-Ca pyroxene exposures as observed by the Moon Mineralogy Mapper (M 3 ). J. Geophys. Res. Planets 116 , E00G06 (2011).
Sinha, R. K. et al. Geological characterization of Chandrayaan-2 landing site in the southern high latitudes of the Moon. Icarus 337 , 113449 (2020).
Pike, R. J. Depth/diameter relations of fresh lunar craters: revision from spacecraft data. Geophys. Res. Lett. 1 , 291–294 (1974).
Ivanov, B. A. Size-frequency distribution of small lunar craters: widening with degradation and crater lifetime. Sol. Syst. Res. 52 , 1–25 (2018).
Borg, L. E., Connelly, J. N., Boyet, M. & Carlson, R. W. Chronological evidence that the Moon is either young or did not have a global magma ocean. Nature 477 , 70–72 (2011).
Pernet-Fisher, J. F., Deloule, E. & Joy, K. H. Evidence of chemical heterogeneity within lunar anorthosite parental magmas. Geochim. Cosmochim. Acta 266 , 109–130 (2019).
Gross, J., Treiman, A. H. & Mercer, C. N. M. in Proc. 43rd Lunar and Planetary Science Conference 2306 (Lunar and Planetary Institute, 2012).
Shirley, D. N. A partially molten magma ocean model. J. Geophys. Res. Solid Earth 88 , A519–A527 (1983).
Elardo, S. M., Laneuville, M., McCubbin, F. M. & Shearer, C. K. Early crust building enhanced on the Moon’s nearside by mantle melting-point depression. Nat. Geosci. 13 , 339–343 (2020).
Gross, J., Treiman, A. H. & Mercer, C. N. Lunar feldspathic meteorites: constraints on the geology of the lunar highlands, and the origin of the lunar crust. Earth Planet. Sci. Lett. 388 , 318–328 (2014).
Song, E., Bandfield, J. L., Lucey, P. G., Greenhagen, B. T. & Paige, D. A. Bulk mineralogy of lunar crater central peaks via thermal infrared spectra from the Diviner Lunar Radiometer: a study of the Moon’s crustal composition at depth. J. Geophys. Res. Planets 118 , 689–707 (2013).
Elkins-Tanton, L. T. Magma oceans in the inner solar system. Annu. Rev. Earth Planet. Sci. 40 , 113–139 (2012).
Chowdhury, A. R. et al. Orbiter high resolution camera onboard Chandrayaan-2 orbiter. Curr. Sci. 118 , 560–565 (2020).
Shearer, C. K., Elardo, S. M., Petro, N. E., Borg, L. E. & McCubbin, F. M. Origin of the lunar highlands Mg-suite: an integrated petrology, geochemistry, chronology, and remote sensing perspective. Am. Mineral. 100 , 294–325 (2015).
Arnaud, K. A., George, I. M. & Tennant, A. F. The OGIP spectral file format. https://heasarc.gsfc.nasa.gov/docs/heasarc/ofwg/docs/spectra/ogip_92_007.pdf (2021).
Arnaud, K. A. in Astronomical Data Analysis Software and Systems V (eds Jacoby, G. H. & Barnes, J.) 17 (Astronomical Society of the Pacific, 1996).
Arnaud, K., Dorman, B., Gordon, C. & Rutkowski, K. Xspec users’ guide. https://heasarc.gsfc.nasa.gov/xanadu/xspec/manual/manual.html (2024).
Campbell, J. L. et al. Calibration of the Mars Science Laboratory alpha particle X-ray spectrometer. Space Sci. Rev. 170 , 319–340 (2012).
Narendranath, S. et al. Lunar elemental abundances as derived from Chandrayaan-2. Icarus 410 , 115898 (2024).
Bhatt, M., Wöhler, C., Grumpe, A., Hasebe, N. & Naito, M. Global mapping of lunar refractory elements: multivariate regression vs. machine learning. Astron. Astrophys. 627 , A155 (2019).
Pillai, N. S. et al. Chandrayaan-2 Large Area Soft X-ray Spectrometer (CLASS): calibration, in-flight performance and first results. Icarus 363 , 114436 (2021).
Taylor, S. R. et al. Composition of the Descartes region, lunar highlands. Geochim. Cosmochim. Acta 37 , 2665–2683 (1973).
Korotev, R. L. in Proc. 12th Lunar and Planetary Science Conference 577–605 (Pergamon Press, 1982).
Laul, J. C. & Schmitt, R. A. in Proc. 4th Lunar and Planetary Science Conference 460 (Pergamon Press, 1973).
Dowty, E., Keil, K. & Prinz, M. in The Apollo 15 Lunar Samples 62–66 (Lunar Science Institute, 1972).
Haskin, L. A., Helmke, P. A., Blanchard, D. P., Jacobs, J. W. & Telander, K. in Proc. 4th Lunar and Planetary Science Conference 1275–1296 (Pergamon Press, 1973).
Haskin, L. A. et al. in Proc. 5th Lunar and Planetary Science Conference 1213–1225 (Pergamon Press, 1974).
Haskin, L. A. & Korotev, R. L. in Proc. 12th Lunar and Planetary Science Conference 404–405 (Pergamon Press, 1981).
Laul, J. C. & Schmitt, R. A. in Proc. 6th Lunar and Planetary Science Conference 1231–1254 (Pergamon Press, 1975).
Philpotts, J. A. et al. in Proc. 4th Lunar and Planetary Science Conference 1427 (Pergamon Press, 1973).
Rhodes, J. M. & Hubbard, N. J. in Proc. 4th Lunar and Planetary Science Conference 1127 (Pergamon Press, 1973).
Ridley, W. I., Hubbard, N. J., Rhodes, J. M., Weismann, H. & Bansal, B. The petrology of lunar breccia 15445 and petrogenetic implications. J. Geol. 81 , 621–631 (1973).
Rose, H. J. Jr et al. in Proc. 4th Lunar and Planetary Science Conference 1149 (Pergamon Press, 1973).
Rose, H. J. Jr et al. in Proc. 6th Lunar and Planetary Science Conference 1363–1373 (Pergamon Press, 1975).
Taylor, S. R. Chemical evidence for lunar melting and differentiation. Nature 245 , 203–205 (1973).
Taylor, S. R. et al. in Proc. 5th Lunar and Planetary Science Conference 789 (Pergamon Press, 1974).
Wänke, H. et al. in Proc. 4th Lunar and Planetary Science Conference 1461 (Pergamon Press, 1973).
Wänke, H. et al. in Proc. 6th Lunar and Planetary Science Conference 1313–1340 (Pergamon Press, 1975).
Wänke, H. et al. in Proc. 7th Lunar and Planetary Science Conference 3479–3499 (Pergamon Press, 1976).
Nava, D. F. in Proc. 5th Lunar and Planetary Science Conference 1087–1096 (Pergamon Press, 1974).
Rhodes, J. M. et al. in Proc. 5th Lunar and Planetary Science Conference 1097–1117 (Pergamon Press, 1974).
Winzer, S. R. et al. Major, minor and trace element abundances in samples from the Apollo 17 station 7 boulder: implications for the origin of early lunar crustal rocks. Earth Planet. Sci. Lett. 23 , 439–444 (1974).
Blanchard, D. P. et al. in Proc. 6th Lunar and Planetary Science Conference 2321–2341 (Pergamon Press, 1975).
Dixon, J. R. & Papike, J. J. in Proc. 6th Lunar and Planetary Science Conference 263–291 (Pergamon Press, 1975).
Dymek, R. F., Albee, A. L. & Chodos, A. A. in Proc. 6th Lunar and Planetary Science Conference 301–341 (Pergamon Press, 1975).
Warner, J. L., Simonds, C. H. & Phinney, W. C. in Proc. 7th Lunar and Planetary Science Conference 915 (Pergamon Press, 1976).
Lindstrom, M. M., Nielsen, R. L. & Drake, M. J. in Proc. 8th Lunar and Planetary Science Conference 2869–2888 (Pergamon Press, 1977).
Lindstrom, M. M., Marvin, U. B., Vetter, S. K. & Shervais, J. W. in Proc. 18th Lunar and Planetary Science Conference 169–185 (Cambridge Univ. Press/Lunar and Planetary Institute, 1988).
Murali, A. V., Ma, M. S., Laul, J. C. & Schmitt, R. A. in Proc. 8th Lunar and Planetary Science Conference 700 (Pergamon Press, 1977).
Warren, P. H. & Wasson, J. T. in Proc. 8th Lunar and Planetary Science Conference 2215–2235 (Pergamon Press, 1977).
Warren, P. H. & Wasson, J. T. in Proc. 9th Lunar and Planetary Science Conference 185–217 (Pergamon Press, 1978).
Warren, P. H. & Wasson, J. T. in Proc. 10th Lunar and Planetary Science Conference 2051–2083 (Pergamon Press, 1979).
Stöeffler, D., Knoell, H. D., Marvin, U. B., Simonds, C. H. & Warren, P. H. in Proc. Conf. Lunar Highlands Crust (eds Merrill, R. B. & Papike, J. J.) 51–70 (Pergamon Press, 1980).
James, O. B. & McGee, J. J. in Proc. 10th Lunar and Planetary Science Conference 713–743 (Pergamon Press, 1979).
Marvin, U. B. & Warren, P. H. in Proc. 11th Lunar and Planetary Science Conference 507–521 (Pergamon Press, 1980).
Ryder, G., Norman, M. D. & Score, R. A. in Proc. 11th Lunar and Planetary Science Conference 471–479 (Pergamon Press, 1980).
Taylor, G. J., Warner, R. D., Keil, K., Ma, M. S. & Schmitt, R. A. in Proc. Conf. Lunar Highlands Crust (eds Merrill, R. B. & Papike, J. J.) 339–352 (Pergamon Press, 1980).
Blanchard, D. P. & McKay, G. A. in Proc. 12th Lunar and Planetary Science Conference 83–85 (Pergamon Press, 1981).
Simonds, C. H. & Warner, J. L. in Proc. 12th Lunar and Planetary Science Conference 993–995 (Pergamon Press, 1981).
Warren, P. H., Taylor, G. J., Keil, K., Marshall, C. & Wasson, J. T. in Proc. 12th Lunar and Planetary Science Conference 1154–1156 (Pergamon Press, 1981).
Warren, P. H., Taylor, G. J., Keil, K., Marshall, C. & Wasson, J. T. in Proc. 12th Lunar and Planetary Science Conference 21–40 (Pergamon Press, 1982).
Warren, P. H., Taylor, G. J., Keil, K., Shirley, D. N. & Wasson, J. T. Petrology and chemistry of two “large” granite clasts from the moon. Earth Planet. Sci. Lett. 64 , 175–185 (1983).
Warren, P. H. et al. Seventh Foray: Whitlockite-rich lithologies, a diopside-bearing troctolitic anorthosite, ferroan anorthosites, and KREEP. J. Geophys. Res. Solid Earth 88 , B151–B164 (1983).
Warren, P. H., Shirley, D. N. & Kallemeyn, G. W. A potpourri of pristine Moon rocks, including a VHK mare basalt and a unique, augite-rich Apollo 17 anorthosite. J. Geophys. Res. Solid Earth 91 , 319–330 (1986).
Warren, P. H., Jerde, E. A. & Morris, R. V. in Proc. 18th Lunar and Planetary Science Conference 1060, (Cambridge Univ. Press, 1987).
Warren, P. H., Jerde, E. A. & Kallemeyn, G. W. in Proc. 20th Lunar and Planetary Science Conference 31–59 (Lunar and Planetary Institute, 1990).
Warren, P. H., Jerde, E. A. & Kallemeyn, G. W. in Proc. 21st Lunar and Planetary Science Conference 51–61 (Lunar and Planetary Institute, 1991).
Hunter, R. H. & Taylor, L. A. The magma ocean from the Fra Mauro shoreline: an overview of the Apollo 14 crust. J. Geophys. Res. Solid Earth 88 , A591–A602 (1983).
James, O. B. & Flohr, M. K. Subdivision of the Mg-suite noritic rocks into Mg-gabbronorites and Mg-norites. J. Geophys. Res. Solid Earth 88 , A603–A614 (1983).
Marti, K. et al. Pieces of the ancient lunar crust: ages and composition of clasts in consortium breccia 67915. J Geophys. Res. Solid Earth 88 , B165–B175 (1983).
Nord, G. L. Jr & Wandless, M. V. Petrology and comparative thermal and mechanical histories of clasts in breccia 62236. J. Geophys. Res. Solid Earth 88 , A645–A657 (1983).
Shervais, J. W., Taylor, L. A. & Laul, J. C. Ancient crustal components in the Fra Mauro breccias. J. Geophys. Res. Solid Earth 88 , B177–B192 (1983).
Shervais, J. W., Taylor, L. A., Laul, J. C. & Smith, M. R. Pristine highland clasts in consortium breccia 14305: petrology and geochemistry. J. Geophys. Res. Solid Earth 89 , C25–C40 (1984).
Ryder, G. in Workshop on the Geology of the Apollo 15 Landing Site (eds Spudis, P. D. & Ryder, G.) 41–45 (Lunar and Planetary Institute, 1985).
Laul, J. C. Chemistry of the Apollo 12 highland component. J. Geophys. Res. Solid Earth 91 , 241–261 (1986).
James, O. B., Lindstrom, M. M. & Flohr, M. K. Petrology and geochemistry of alkali gabbronorites from lunar breccia 67975. J. Geophys. Res. Solid Earth 92 , E314–E330 (1987).
James, O. B., Lindstrom, M. M. & Flohr, M. K. in Proc. 19th Lunar and Planetary Science Conference 219–243 (Cambridge Univ. Press/Lunar and Planetary Institute, 1989).
Marvin, U. B., Lindstrom, M. M., Bernatowicz, T. J., Podosek, F. A. & Sugiura, N. The composition and history of breccia 67015 from North Ray Crater. J. Geophys. Res. Solid Earth 92 , E471–E490 (1987).
Simon, S. B., Papike, J. J., Laul, J. C., Hughes, S. S. & Schmitt, R. A. Apollo 16 regolith breccias and soils: recorders of exotic component addition to the Descartes region of the moon. Earth Planet. Sci. Lett. 89 , 147–162 (1988).
Ryder, G. & Sherman, S. B. The Apollo 15 coarse fines (4–10 mm). NASA Technical Memorandum 19900005711 (1989).
Lindstrom, M. M., Marvin, U. B. & Mittlefehldt, D. W. in Proc. 19th Lunar and Planetary Science Conference 245–254 (Cambridge Univ. Press/Lunar and Planetary Institute, 1989).
Jolliff, B. L., Korotev, R. L. & Haskin, L. A. in Proc. 21st Lunar and Planetary Science Conference 193–219 (Lunar and Planetary Institute, 1991).
Norman, M. D. & Taylor, S. R. Geochemistry of lunar crustal rocks from breccia 67016 and the composition of the Moon. Geochim. Cosmochim. Acta 56 , 1013–1024 (1992).
Snyder, G. A., Taylor, L. A., Liu, Y. G. & Schmitt, R. A. in Proc. 22nd Lunar and Planetary Science Conference 399–416 (Lunar and Planetary Institute, 1992).
Shih, C. Y. et al. Ages of pristine noritic clasts from lunar breccias 15445 and 15455. Geochim. Cosmochim. Acta 57 , 915–931 (1993).
Jolliff, B. L. & Haskin, L. A. Cogenetic rock fragments from a lunar soil: evidence of a ferroan noritic-anorthosite pluton on the Moon. Geochim. Cosmochim. Acta 59 , 2345–2374 (1995).
Norman, M. D., Keil, K., Griffin, W. L. & Ryan, C. G. Fragments of ancient lunar crust: petrology and geochemistry of ferroan noritic anorthosites from the Descartes region of the Moon. Geochim. Cosmochim. Acta 59 , 831–847 (1995).
Norman, M. D., Borg, L. E., Nyquist, L. E. & Bogard, D. D. Chronology, geochemistry, and petrology of a ferroan noritic anorthosite clast from Descartes breccia 67215: clues to the age, origin, structure, and impact history of the lunar crust. Meteorit. Planet. Sci. 38 , 645–661 (2003).
Hansen, E. C., Smith, J. V. & Steele, I. M. in Proc. 11th Lunar and Planetary Science Conference 523–533 (Pergamon Press, 1980).
Hollocher, K. NORM4 Excel spreadsheet programs to calculate petrologic norms from whole-rock chemical analyses. Zenodo https://doi.org/10.5281/zenodo.5818037 (2022).
Lucey, P. G., Blewett, D. T., Taylor, G. J. & Hawke, B. R. Imaging of lunar surface maturity. J. Geophys. Res. Planets 105 , 20377–20386 (2000).
McEwen, A. S. in Proc. 27th Lunar and Planetary Science Conference 841 (Lunar and Planetary Institute, 1996).
Pieters, C. M. in Proc. Workshop on New Views of the Moon II: Understanding the Moon Through the Integration of Diverse Datasets 8025 (Lunar and Planetary Institute, 1999).
Download references
Acknowledgements
The APXS experiment is designed and developed by the Physical Research Laboratory (PRL), Ahmedabad, supported by the Department of Space, Government of India. The Space Applications Centre (SAC), Ahmedabad, supported the fabrication and space qualification of the APXS flight model. We acknowledge the extensive support from the entire Chandrayaan-3 team, consisting of large groups from U R Rao Satellite Centre (URSC), Bangalore; Vikram Sarabhai Space Center (VSSC), Thiruvananthapuram; ISRO Telemetry, Tracking and Command Network (ISTRAC), Bangalore; Satish Dhawan Space Centre (SDSC), Sriharikota, as well as other ISRO centres. We specifically acknowledge the efforts of various teams involved in the operations of the Chandrayaan-3 Pragyan rover. We acknowledge the use of images from Chandrayaan-3 rover NavCam developed by the Laboratory for Electro-Optics Systems (LEOS) and processed by the SAC and the use of the image from the Orbiter High Resolution Camera (OHRC) aboard the Chandrayaan-2 orbiter provided by the SAC data processing team and archived at the Indian Space Science Data Center (ISSDC). We also acknowledge the use of data from the Chandrayaan-1 M 3 , LRO WAC, Kaguya TC and Clementine UVVIS instruments. A.B. was J.C. Bose Fellow during this work.
Author information
Authors and affiliations.
Physical Research Laboratory (PRL), Ahmedabad, India
Santosh V. Vadawale, N. P. S. Mithun, M. Shanmugam, Amit Basu Sarbadhikari, Rishitosh K. Sinha, Megha Bhatt, S. Vijayan, Neeraj Srivastava, Anil D. Shukla, S. V. S. Murty, Anil Bhardwaj, Y. B. Acharya, Arpit R. Patel, Hiteshkumar L. Adalaja, C. S. Vaishnava, B. S. Bharath Saiguhan, Nishant Singh, Sushil Kumar, Deepak Kumar Painkra, Yash Srivastava, Varsha M. Nair, Tinkal Ladiya, Shiv Kumar Goyal & Neeraj K. Tiwari
Department of Geology, Hemvati Nandan Bahuguna University, Srinagar Garhwal, India
Anil D. Shukla
U R Rao Satellite Centre (URSC), ISRO, Bangalore, India
Shyama Narendranath, Netra S. Pillai, Abhishek Kumar, Neeraj Satya, Vivek R. Subramanian, Sonal G. Navle, R. G. Venkatesh & Lalitha Abraham
Space Applications Centre (SAC), ISRO, Ahmedabad, India
Arup Kumar Hait, Aaditya Patinge, K. Suresh & Amitabh
You can also search for this author in PubMed Google Scholar
Contributions
S.V.V., M.S., Y.B.A. and S.V.S.M. designed the experiment. M.S., A.R.P., H.L.A., T.L., S.K.G. and N.K.T. developed the APXS instrument. N.P.S.M., N.Si., S.K. and D.K.P. conducted the calibration experiments. A.K.H., A.P., A.K., N.Sa., V.R.S., S.G.N., R.G.V. and L.A. contributed to the mechanical, thermal and mechanism design and integration with the rover. K.S. and Am. provided the rover NavCam and Orbiter High Resolution Camera (OHRC) images. N.P.S.M., C.S.V. and B.S.B.S. carried out data processing. S.V.V. and N.P.S.M. performed the APXS data analysis. A.B.S. and Y.S. carried out the lithological and geochemical analysis. R.K.S., M.B., S.V. and N.Sr. carried out the remote-sensing analysis. A.D.S., A.B.S., Y.S. and V.M.N. carried out laboratory XRF measurements. S.N. and N.S.P. provided remote-sensing XRF data. S.V.V., N.P.S.M., A.B.S., R.K.S., M.B., S.V., N.Sr. and A.B. contributed to the interpretation and preparation of the manuscript.

Corresponding author
Correspondence to Santosh V. Vadawale .
Ethics declarations
Competing interests.
The authors declare no competing interests.
Peer review
Peer review information.
Nature thanks Paul Warren and the other, anonymous, reviewer(s) for their contribution to the peer review of this work. Peer reviewer reports are available.
Additional information
Publisher’s note Springer Nature remains neutral with regard to jurisdictional claims in published maps and institutional affiliations.
Extended data figures and tables
Extended data fig. 1 maps of elemental abundances derived from m 3 ..
Elemental abundance maps derived from M 3 observations around the landing site (1° × 1°) are shown for Mg ( a ), Fe ( b ) and Ca ( c ). The blue square represents the landing site. Typical uncertainties in abundances are 1 wt% and the colour scale spans about 2 σ around the value at the landing site. This shows that there is no variation in abundance over this spatial scale.
Extended Data Fig. 2 Reflectance spectrum of the landing site region.
a , M 3 albedo map at 1,578 nm around the Chandrayaan-3 landing site. This 1° × 1° subset was extracted from M 3 product ID M3G20090606T010302. The spatial resolution of the M 3 strip used here is 280 m pixel −1 . The blue dot in the centre shows the landing site. b , Extracted M 3 spectrum from the pixel containing the Chandrayaan-3 landing site (marked by the blue dot in panel a ). This reflectance spectrum represents a highland-type soil spectrum with no notable absorption feature at 1 and 2 μm.
Extended Data Fig. 3 Source of the material at the landing site.
a , Kaguya TC orthoimage showing the region around the landing site (marked by the solid yellow circle). The chains and clusters of secondaries (classes 1 and 2) are marked in the figure. Solid white lines represent the boundary of the ‘Ntp’ geological unit (intercrater plains region). b , False-colour composite image of band ratios from Clementine UVVIS 134 . The colours correspond to band ratios of 750 nm/415 nm (red), 750 nm/950 nm (green) and 415 nm/750 nm (blue). In the figure, mature lunar highlands appear in shades of red and the bright blue shades represent rays of a young crater 135 , 136 . Arrows mark the ejecta rays from the Schomberger crater extending towards the landing site.
Extended Data Fig. 4 APXS spectrum fitted with the model showing different components.
APXS spectrum (blue data points) from observation ID 18 fitted with the model consisting of continuum component (dashed black line) and lines of different elements. Dashed lines of different colours denote the line components corresponding to the elements marked with the same colour.
Extended Data Fig. 5 Distance dependence of line intensity and distance estimation from continuum rate.
a , Slope of the line intensity–abundance correlation, which is wt% per unit photons per second, of different elements as a function of the sample distance. Data points are the slope values; the solid lines show the power laws that fit them. b , Continuum rates (sum of count rates in 22.4–24.2-keV energy range) obtained for different geochemical reference materials are shown as a function of distance with the red data points and the black line shows the best-fit model. The continuum rate from lunar observation for observation ID 18, along with its error, is plotted on the curve to estimate the respective distance. The blue-shaded regions show uncertainties in the continuum rate and corresponding uncertainty in distance estimates. Uncertainties in the estimated distance are then propagated to abundance measurements through correlation parameters.
Extended Data Fig. 6 Abundance measurement from correlations.
An example of abundance measurement of the lunar sample (observation ID 15) from correlations obtained from ground calibration is shown. Dashed lines and faint points are correlations from USGS samples shown in Supplementary Figs. 2 and 3 . Vertical grey shades show the measured line intensity with error from the lunar observation. Three solid lines show the correlation for the measured distance (orange) and at one standard deviation of the distance (blue and green). Matrix-corrected flux values are plotted on these correlations to obtain the abundances (error bars with respective colours). Measured abundance is shown with the red star symbols and associated uncertainties, including the distance uncertainties, are also shown.
Extended Data Fig. 7 Coadded spectrum from all observations.
APXS spectrum obtained by adding data from all 23 scientific observations amounting to a total exposure of 31.34 h. An extra line of zinc (K α ) is detected in the coadded spectrum.
Supplementary information
Supplementary information.
The Supplementary Information file includes a supplementary section on calibration, Supplementary Figs. 1–4 and Supplementary Tables 1–5.
Peer Review File
Source data, source data fig. 2, source data fig. 3, rights and permissions.
Springer Nature or its licensor (e.g. a society or other partner) holds exclusive rights to this article under a publishing agreement with the author(s) or other rightsholder(s); author self-archiving of the accepted manuscript version of this article is solely governed by the terms of such publishing agreement and applicable law.
Reprints and permissions
About this article
Cite this article.
Vadawale, S.V., Mithun, N.P.S., Shanmugam, M. et al. Chandrayaan-3 APXS elemental abundance measurements at lunar high latitude. Nature 633 , 327–331 (2024). https://doi.org/10.1038/s41586-024-07870-7
Download citation
Received : 02 January 2024
Accepted : 23 July 2024
Published : 21 August 2024
Issue Date : 12 September 2024
DOI : https://doi.org/10.1038/s41586-024-07870-7
Share this article
Anyone you share the following link with will be able to read this content:
Sorry, a shareable link is not currently available for this article.
Provided by the Springer Nature SharedIt content-sharing initiative
Quick links
- Explore articles by subject
- Guide to authors
- Editorial policies
Sign up for the Nature Briefing newsletter — what matters in science, free to your inbox daily.

- The Magazine
- Stay Curious
- The Sciences
- Environment
- Planet Earth
The Chandrayaan-3 Mission and the Second Space Race to the Moon’s Dark Side
India’s landmark 2023 mission to the lunar south pole revealed the moon may be more habitable than we think..
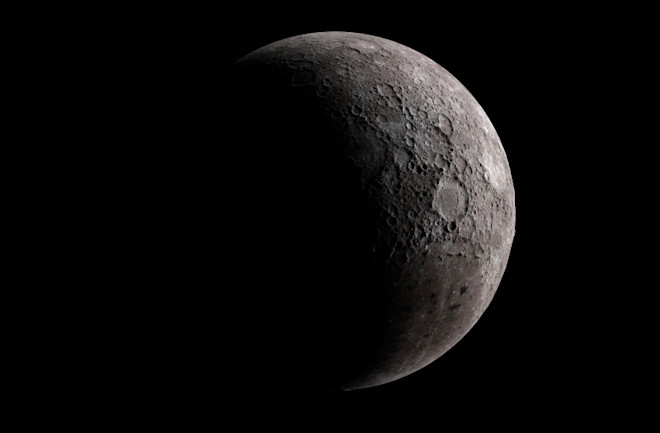
Humanity has long been captivated by the dark side of the moon, known for its mystery and opportunities for discovery. For decades, though, the moon’s darkest and most distant regions were thought to be unreachable.
But in August 2023, the Indian Space Research Organization (ISRO) launched the Chandrayaan-3 mission, which became the first spacecraft to land near the moon’s south pole.
Outfitted with groundbreaking temperature detection and chemical analysis technology, Chandrayaan-3 collected the first ever on-the-ground observations of the southernmost lunar surface. Its findings revealed that, amid the darkness of the moon’s poles, there exists the possibility of human survival on the moon, propelling forward human interest in establishing a habitable lunar colony.
Searching for Ice Beneath the Lunar’s Surface
Ever since Apollo 11 brought the first astronauts to the moon in 1969, most lunar landings have centered around the moon’s equator. But the moon’s North and South poles have long fascinated experts, mainly because they’re some of the most likely places on the moon where water ice could exist.
For decades, scientists have searched for water on the moon, in hopes of finding a way to make the harsh environment habitable for humans. But water can’t exist in its liquid form in space — if it does exist on the moon, it’s present either as water vapor, or as ice, frozen beneath the lunar surface.
The moon’s South Pole is home to some of the solar system’s largest, most ancient craters, and because of the angle at which sunlight hits the moon’s surface, most of this polar region hasn’t seen sunlight in billions of years.
While these craters’ immense size, freezing temperatures, and permanent darkness makes them difficult landing sites, it’s these same traits that give them a high potential for holding deposits of water ice. ISRO’s Chandrayaan-1 mission in 2018 confirmed the existence of water ice deposit near the moon’s poles , and experts have since sought to further explore this region and analyze these deposits.
Reaching the moon’s polar extremes is no easy feat — just days before the Chandrayaan-3 mission landed near the lunar South pole, Russia’s Luna-25 mission crashed while trying to reach another polar landing site.
But if water ice is discovered at these sites, it could help sustain astronauts during longer moon missions, and could even be used as drinking water at future lunar colonies.
Read More: The Search for Ice Deposits in Moon Craters
Discovery of Sulfur on the Moon
After the Chandrayaan-3 mission successfully landed near the lunar South Pole on August 22, 2023, the spacecraft’s Vikram rover spent about 10 days exploring the area, conducting the first ever on-the-ground tests of the lunar South Pole’s surface.
For instance, tests conducted on lunar surface composition provided the first confirmation of sulfur on the moon’s surface. Previous moon missions and orbiters had discovered sulfur in tiny amounts, mostly in lunar rocks and minerals.
But the Chandrayaan-3’s Vikram rover was the first to measure the elemental composition of the South Pole directly on the surface, and its laser technology allowed it to provide the most accurate possible measurements, according to a release from the ISRO.
This discovery could pave the way for on-site space station construction in the future: sulfur can be used to create solar cells, fertilizer, and even concrete.
What’s more, higher-than-expected sulfur concentrations could indicate the presence of nearby water ice.
“The sulfur measurement is intriguing because sulfur may point toward the source of the Moon’s water. So, scientists can use temperature as a way of finding where volatiles like these may end up,” writes Paul Hayne of the University of Colorado-Boulder in a November 2023 article in the university’s Arts and Sciences Magazine .
Read More: Earth's Moon: The Basics of its Origin, Evolution and Exploration
Lunar Soil Is a Powerful Insulator
The mission’s Vikram rover also came equipped with a temperature probe, which collected samples that extended 10 centimeters below the surface of the moon and analyzed their temperatures.
The results: Scientists found that just 8 cm below the surface, the soil was nearly 60 degrees Celsius cooler than it was on the surface.
As the first ever in-situ temperature measurements taken at the moon’s south pole, the discovery revealed that lunar soil is a powerful insulator. Like the discovery of sulfur, this could pave the way for on-site construction projects using soil as insulation.
And with significantly cooler temperatures beneath the surface, it could also indicate the presence of water ice further below. In space, water converts from ice to water vapor at an extremely low temperature of negative 160 degrees Celsius, but powerful insulation from lunar soil could enable water ice deposits to remain in their solid form underground or in deep, shadowed craters.
Read More: Scientists Grow First Plants in Lunar Soil
A Second Space Race
After successfully completing experiments and collecting data on the lunar surface, the Chandrayaan-3 propulsion module was originally intended to remain in orbit around the moon, conducting further observations from afar.
However, the ISRO announced in December 2023 that the spacecraft had used its leftover fuel to re-enter high Earth orbit in November, where it remains today to conduct additional experiments. The propulsion module will remain in orbit for at least one year, though the ISRO has not shared its plans for when the spacecraft runs out of fuel.
Meanwhile on the ground, Chandrayaan-3’s discoveries have prompted a renewed interest in space travel to the moon. NASA is currently planning its Artemis III mission, which aims to send the first humans to explore the lunar south pole as soon as September 2026. China’s Chang’e-7 and Chang’e-8 missions, set to launch in 2026 and 2028 respectively, are also both shooting for the south pole.
With its promise for habitability and unexpectedly robust resources, the lunar south pole has sparked what some experts are calling a “second space race.” As governmental and commercial missions alike seek out water on the dark side of the moon, one thing is certain: the next decade could bring a host of new lunar discoveries, and even a lunar space station.
Read More: Here Are 4 Reasons Why We Are Still Going to the Moon
Article Sources
Our writers at Discovermagazine.com use peer-reviewed studies and high-quality sources for our articles, and our editors review for scientific accuracy and editorial standards. Review the sources used below for this article:
NASA. Water & Ices on the Moon
University of Colorado Boulder. Colorado Arts and Sciences Magazine
ISRO. Returns to home Earth: Chandrayaan-3 Propulsion Module moved from Lunar orbit to Earth's orbit
- human spaceflight
- space exploration
- spaceflight
Already a subscriber?
Register or Log In
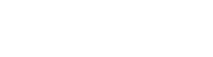
Keep reading for as low as $1.99!
Sign up for our weekly science updates.
Save up to 40% off the cover price when you subscribe to Discover magazine.


IMAGES
VIDEO
COMMENTS
Here are the list of findings made on the Moon's surface so far: 1. Temperature recorded on the Lunar surface: On 27 August, ISRO released a graph of the temperature variation on the lunar surface...
Chandrayaan-3 experiments and beyond. Timeline. 27th August 2023: LM ChaSTE (Chandra’s Surface Thermo-physical Experiment) instrument makes its first observations, measuring the temperature profile of the lunar regolith around the South Pole to gain understanding of the thermal behaviour of the lunar surface.
Chandrayaan-3 will perform a raft of experiments, including a spectrometer analysis of the mineral and chemical composition of the Moon's surface, returning valuable data on the properties of...
Chandrayaan-3 ( CHUN-drə-YAHN) is the third mission in the Chandrayaan programme, a series of lunar-exploratio / ˌ tʃ ʌ n d r ə ˈ j ɑː n / n missions developed by the Indian Space Research Organisation (ISRO). [11] The mission consists of a Vikram lunar lander and a Pragyan lunar rover was launched from Satish Dhawan Space Centre on 14 ...
Chandrayaan-3 is a follow-on mission to Chandrayaan-2 to demonstrate end-to-end capability in safe landing and roving on the lunar surface. It consists of Lander and Rover configuration. It will be launched by LVM3 from SDSC SHAR, Sriharikota.
The three main objectives of Chandrayaan-3 are to land safely on the surface, to demonstrate rover operations and to perform scientific experiments on site, according to the official website.
Here we report the first in situ measurements of the elemental abundances in the lunar southern high-latitude regions by the Alpha Particle X-ray Spectrometer (APXS) experiment 10...
Chandrayaan-3 is a follow-on mission to Chandrayaan-2 to demonstrate end-to-end capability in safe landing and roving on the lunar surface. It consisted of a Lander, Rover and Propulsion module to carry the landing module till 100km lunar orbit.
The results: Scientists found that just 8 cm below the surface, the soil was nearly 60 degrees Celsius cooler than it was on the surface. As the first ever in-situ temperature measurements taken at the moon’s south pole, the discovery revealed that lunar soil is a powerful insulator.
When a laser detector mounted on the rover measured the chemicals present on the lunar surface near the south pole, it found a host of chemicals such as aluminium, calcium, iron, chromium,...