Advertisement

Islet autoimmunity in human type 1 diabetes: initiation and progression from the perspective of the beta cell
- Invited Review
- Open access
- Published: 25 July 2023
- Volume 66 , pages 1971–1982, ( 2023 )
Cite this article
You have full access to this open access article
- Peter J. Thompson ORCID: orcid.org/0000-0002-6851-8899 1 , 2 ,
- Jasmine Pipella ORCID: orcid.org/0009-0005-9936-8386 1 , 2 ,
- Guy A. Rutter ORCID: orcid.org/0000-0001-6360-0343 3 , 4 , 5 ,
- Herbert Y. Gaisano ORCID: orcid.org/0000-0001-5213-9168 6 &
- Pere Santamaria ORCID: orcid.org/0000-0003-3469-1586 7 , 8
8262 Accesses
6 Citations
60 Altmetric
Explore all metrics
Type 1 diabetes results from the poorly understood process of islet autoimmunity, which ultimately leads to the loss of functional pancreatic beta cells. Mounting evidence supports the notion that the activation and evolution of islet autoimmunity in genetically susceptible people is contingent upon early life exposures affecting the islets, especially beta cells. Here, we review some of the recent advances and studies that highlight the roles of these changes as well as antigen presentation and stress response pathways in beta cells in the onset and propagation of the autoimmune process in type 1 diabetes. Future progress in this area holds promise for advancing islet- and beta cell-directed therapies that could be implemented in the early stages of the disease and could be combined with immunotherapies.
Graphical Abstract
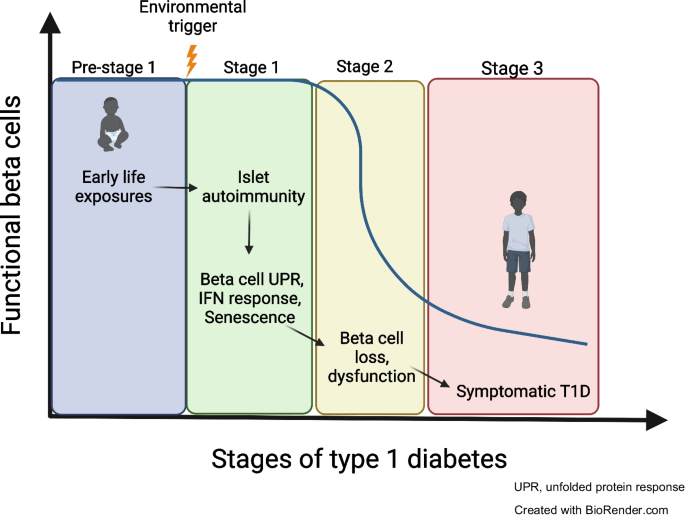
Similar content being viewed by others
Immunobiology of β-Cell Destruction
Pathogenesis of Type 1 Diabetes
Avoid common mistakes on your manuscript.
Introduction
Type 1 diabetes results from organ-specific autoimmunity, which eliminates most of the insulin-producing pancreatic beta cells. While the role of islet autoimmunity remains unquestioned, the role of the islets as active participants has only recently become accepted [ 1 ]. It was once believed that virtually all beta cells are lost in type 1 diabetes. However, it now is clear that there is a range of residual beta cells that are dysfunctional in people with newly diagnosed type 1 diabetes [ 2 ]. In addition, alpha cell dysfunction is recognised as a prominent feature of the disease [ 3 ].
Undoubtedly, sustained efforts by the global type 1 diabetes research community to understand the basis of T cell-driven autoimmunity in type 1 diabetes have paid dividends. The first US Food and Drug Administration-approved immunotherapy for type 1 diabetes, the anti-CD3 antibody teplizumab, delays symptomatic type 1 diabetes onset by 2–3 years [ 4 ], opening the door for the future clinical application of antigen-specific approaches with potentially superior immunoregulatory properties [ 5 ]. Notwithstanding this achievement, we are still far from a nuanced understanding of how islet autoimmunity originates and propagates during the development of type 1 diabetes and why it is so heterogeneous among individuals. In NOD mice, the following observations have been made: development of type 1 diabetes requires CD4 + and CD8 + T cells; autoreactive T cells differentiate into killers by engaging beta cell antigens on local antigen-presenting cells; initiating CD4 + T cells are insulin-reactive; and CD8 + T cells play a major role as beta cell killers [ 6 , 7 ]. Immunohistopathological studies of pancreases from individuals with type 1 diabetes are generally compatible with these observations made in mice (see references below) but have exposed additional phenomena peculiar to the natural history of the disease in humans. In particular, the dialogue between T cells and beta cells is far more complex in humans and recent evidence supports potential type 1 diabetes ‘endotypes’ based on age at onset [ 8 , 9 ]. Here, we draw upon studies that have shed light on the initiation and progression of islet autoimmunity from the point of view of the beta cell. We advance the hypothesis that antigen presentation by beta cells, their stress responses and functional heterogeneity are critical factors that will provide clues towards solving the enigma posed by islet autoimmunity in type 1 diabetes.
How is islet autoimmunity initiated? The chicken and the egg
Autoantibody positivity.
Central to solving the puzzle as to how type 1 diabetes occurs will be to identify the elusive cause(s) of initial islet autoimmunity. The concept that islet autoimmunity results from genetic susceptibility combined with an environmental trigger, first theorised by Eisenbarth [ 10 ], is now generally accepted [ 11 ]. Symptomatic, or ‘stage 3’ type 1 diabetes, generally occurs following two clinically tractable stages consisting of seroconversion and the presence of two or more autoantibodies, followed by development of dysglycaemia [ 11 ]. While valuable clinically, the model has some limitations, some of which are just emerging. For example, the single-autoantibody-positive stage generally has a significantly lower of risk of progression [ 12 ] and as such is not considered as the earliest stage of type 1 diabetes. Nevertheless, recent work has revealed defects in glucagon secretion in islets isolated from donors positive for a single GAD autoantibody (GADA) [ 13 ]. Furthermore, all autoantibodies are not equal in terms of risk, and the order of autoantibody appearance influences progression to type 1 diabetes [ 14 ]: 22% of single-GADA-positive individuals progress to multiple autoantibodies; and about 6% of those with multiple antibodies progress to stage 3 symptomatic type 1 diabetes [ 15 ]. On the other hand, there is heterogeneity between those individuals that develop multiple autoantibodies, with many not progressing to type 1 diabetes [ 16 ]. Regardless, in terms of initiation of islet autoimmunity, the model posits an environmental triggering event of some kind.
Benign autoimmunity
Although insulin is only one of many type 1 diabetes-relevant autoantigens, several lines of evidence suggest that insulin autoreactivity, both in mice and humans, plays a key role in the initiation of type 1 diabetes [ 17 , 18 ]. Mounting evidence also suggests that diabetogenesis involves CD4 + T cell recognition of hybrid peptides generated by fusion of insulin to other autoantigens such as chromogranin A and islet amyloid polypeptide (hybrid insulin peptides [HIPs]) [ 19 ]. Although it remains unclear why and how these T cells become activated, an extra-pancreatic priming event triggered by systemic delivery of the beta cell-generated insulin peptides is a likely possibility [ 20 ]. In turn, it seems reasonable to propose that priming of the disease-initiating T cell specificities is preceded by quantitatively and/or qualitatively abnormal exposure of these T cells to cognate insulin epitopes.
In agreement with these considerations, a growing body of evidence supports the view that most if not all people harbour autoreactive T cells in the peripheral repertoire, regardless of their autoimmune disease proclivity [ 21 ]. While this is inconsequential in most individuals, beta cell insults coupled to genetic predisposition could trigger the activation and recruitment of these T cells to target organs, such as the pancreatic islets in type 1 diabetes. For example, CD8 + T cells recognising epitopes from several beta cell autoantigens circulate at similar frequencies in both individuals with type 1 diabetes and non-diabetic individuals but are exclusively recruited to the pancreas in individuals with type 1 diabetes, where they exhibit an antigen-experienced memory phenotype [ 22 ]. Coppieters et al [ 23 ] demonstrated the presence of various beta cell autoreactive CD8 + T cell specificities in pancreases from a subset of individuals up until 8 years post diagnosis, highlighting the chronic and heterogeneous nature of the autoimmune attack underlying the progression of type 1 diabetes. In a similar study, Bender et al [ 24 ] used in situ tetramer staining to demonstrate that preproinsulin-specific CD8 + T cells are found in healthy donor pancreas tissue in similar proportions to those in autoantibody-positive stage 1–2 donors and donors with recent-onset type 1 diabetes. In healthy donor pancreases, these self-reactive CD8 + T cells appear to remain in a seemingly dormant state, dispersed in the exocrine compartment. However, in pancreases from donors with type 1 diabetes, these CD8 + T cells are much more frequently observed in contact and proximity with insulin-containing islets and display features of antigen experience [ 24 ]. Similarly, a higher frequency of circulating preproinsulin-specific CD8 + T cells exhibit an antigen-experienced memory phenotype in recent-onset type 1 diabetes and in stage 2 autoantibody-positive children, as compared with healthy control individuals [ 25 ]. Together, these studies suggest that initiation of islet autoimmunity may require beta cell insults capable of eliciting the activation and recruitment of autoreactive T cells to the pancreas. Genetically imprinted inter-individual differences in the avidity of specific autoreactive T cells, differences in absolute autoreactive T cells frequencies, and/or differences in T cell activation thresholds, which are impacted by thymic selection processes, can help determine the likelihood and the extent to which one or more ‘environmental’ triggers’ may lead to chronic autoimmunity and eventually beta cell destruction [ 26 ].
Environmental triggers
Putative environmental triggers for islet autoimmunity in type 1 diabetes remain elusive (reviewed in [ 27 ]) but molecular mimicry between beta cell and viral antigens [ 22 , 28 ] may be a plausible link that explains the selective activation of islet-reactive T cells in certain individuals. Epidemiological studies of large prospective paediatric cohorts have provided insights into early life exposures associated with seroconversion, progression to multiple autoantibodies and finally symptomatic type 1 diabetes [ 29 , 30 ]. The BABYDIAB and BABYDIET studies established that in genetically susceptible newborns the peak incidence of seroconversion (development of the first islet autoantibody) occurs between 9 months and 2 years of age [ 29 ], and children who develop multiple autoantibodies before age 3 years are much more likely to progress to type 1 diabetes in the ensuing 10 years as compared with those who do not [ 30 ]. Recent updates from The Environmental Determinants of Diabetes in the Young (TEDDY) study support the view that the development of islet autoimmunity is associated with chronic enteroviral B infection [ 31 ]. New findings from the Diabetes Virus Detection (DiViD) study lend further evidence implicating live enteroviruses in new-onset type 1 diabetes [ 32 ]. In the first study of its kind to demonstrate live virus in the pancreas of living individuals with type 1 diabetes, the authors found that resected pancreas tail tissue from 6/6 individuals recently diagnosed with type 1 diabetes (within 1 month of diagnosis) harboured enterovirus, whereas only two out of 11 from normoglycaemic/non-diabetic individuals did [ 32 ]. Remarkably, other viruses were much less frequently found in the diabetic pancreases [ 32 ], arguing against a general predisposition to viral infection in people with type 1 diabetes. While a potential environmental triggering event could plausibly consist of persistent enteroviral infection, it is important to note that previous studies have not found a correlation between enteroviruses and type 1 diabetes (extensively reviewed in [ 33 ]) and it is unlikely that the ensuing heterogeneity in the progression of islet autoimmunity could be explained by a single kind of environmental trigger in all cases. Future investigations on individuals at early presymptomatic stages of type 1 diabetes will be required to establish whether chronic enteroviral infections could selectively promote activation of beta cell-specific T cell populations. Additional studies should also consider events even earlier in life, such as in utero sensitising events, and their impact during the window of beta cell development in the fetus. Indeed, recent evidence from the TEDDY study showed a correlation between the number and type of islet autoantibody and various forms of maternal psychosocial stress during pregnancy [ 34 ].
Early life metabolic changes
But is islet autoimmunity merely a matter of immune-activating events or do earlier clinically tractable events occur in the islets/beta cells? A recent study by Warncke et al [ 35 ] sheds light on this vital question for the first time through a comprehensive analysis of pre- and postprandial glucose levels in a large cohort of infants prior to seroconversion within the critical window of islet autoimmunity development. This study analysed over 5000 preprandial and over 3000 postprandial blood glucose measurements in a prospective cohort of over 1000 infants and toddlers from 4 months to 3.5 years of age as part of the Primary Oral Insulin Trial (POInT) [ 36 ]. Remarkably, the study demonstrated that there are subtle but significant increases in 30 min postprandial blood glucose in infants (from 4 months to 1.5 years of age) that experienced seroconversion to single or multiple autoantibodies as compared with those that did not [ 35 ]. At toddler stages (1.5–3.5 years) differences were observed in preprandial blood glucose between the groups. Seroconversion was detected at a median age of 1.8 years but postprandial blood glucose levels did not differ between infants that went on to develop islet autoantibodies vs those that did not, just up until 2 months prior to seroconversion [ 35 ]. Strikingly, these data suggest that alterations in glucose metabolism and/or beta cell function precede the development of islet autoimmunity [ 35 ] and it is tempting to speculate that early life alterations in beta cell function could play a causal role in islet autoimmunity. There are important limitations to consider in this study, since at present it is unclear whether autoimmune initiation is the same as seroconversion. Nevertheless, this study furnishes a new perspective on the role of early postnatal development in shaping the functioning and responsiveness of islets and their interactions with the immune system in humans. A body of evidence from studies in rodents highlights the importance of diet interactions with the epigenome in neonatal beta cells (reviewed in [ 37 ]) and supports the importance of the neonatal period in laying the foundations for the long-term health of beta cells in adults. As very few studies have interrogated the mechanisms of human beta cell development and function in the neonatal period [ 38 , 39 , 40 ], further work in this area is urgently needed. Major consortia, such as the US NIH-funded Human Atlas of Neonatal Development & Early Life Pancreas (HANDEL-P) ( https://www.pancreatlas.org/datasets/531/overview , accessed 23 June 2023), have the resources to make advances in this field on behalf of the community.
How does islet autoimmunity progress? A role for antigen presentation by beta cells
Beta cells and the progression of islet autoimmunity.
The initiation of islet autoimmunity is likely to involve both early life developmental changes in beta cells as well as environmental exposures but what factors drive the further progression and development of islet autoimmunity? Islet autoimmunity dynamically evolves and expands during the progression from stage 1 to stage 3 type 1 diabetes, as evidenced by the presence of increasing numbers of autoantibodies and autoreactive T cells specificities. Islet-reactive T cells recognise an increasing number of beta cell antigens and epitopes as the disease progresses, consistent with both antigen and epitope spreading [ 41 ]. Thus, dynamic antigen presentation by beta cells may be a driving factor in the progression of islet autoimmunity (reviewed in [ 42 ]). It is well established that human islets overexpress HLA Class I molecules during early stages of type 1 diabetes [ 43 ]; however, studies now report expression of HLA Class II molecules on beta cells in type 1 diabetes as well [ 44 , 45 ]. Elegant immunopeptidomics of HLA Class I from human beta cell lines and tetramer staining confirms that beta cells present both conventional and modified antigens to islet-infiltrating CD8 + T cells [ 46 , 47 ]. Likewise, studies of human islet-reactive CD4 + T cells have shown that at least some of the beta cell-derived epitopes that are targeted by diabetogenic CD4 + T cells arise from alternative splicing of antigen-coding mRNAs or from the post-translational fusion of fragments of insulin and other secretory granule hormones, the so-called HIPs [ 48 , 49 ]. T cells recognising these antigenic targets would readily escape central tolerance, and hence play a more significant, albeit not exclusive, role in diabetogenesis owing to their higher avidity for cognate peptide major histocompatibility complex (pMHC) (in the absence of thymic censorship). One of the main take-home messages from this body of work is that conventional and typically processed autoantigens only represent the tip of the iceberg in terms of antigen complexity during the progression of islet autoimmunity. Preproinsulin and insulin (or derivatives) are major antigenic targets of the human autoreactive T cell repertoire that infiltrate islets in type 1 diabetes [ 17 , 50 ]. Post-translational modification, alternative splicing, hybrid-fusion, defective ribosomal products and other unconventional beta cell antigens have all been shown to underlie the activation of at least some autoreactive T cells responses. These unconventional beta cell autoantigens are collectively referred to as ‘neoantigens’ (reviewed extensively elsewhere [ 28 ]), since they comprise immunologically novel sequences or structures that are selectively expressed (and exposed to autoreactive T cells) in the islet niche. As antigen presentation by beta cells is greatly altered by various forms of stress (reviewed in [ 51 ] and further detailed below), stress pathways operating in beta cells are a major cause of neoantigen formation.
Beta cell death and islet autoimmunity
Another major unanswered question concerns the relationship between beta cell death and islet autoimmunity progression. As the mode and kinetics of beta cell death during the presymptomatic stages of type 1 diabetes clearly vary between people, how this influences the evolution of antigen presentation and neoantigen formation remains to be determined. Neiman et al [ 52 ] measured cell-free beta cell DNA (identified by its beta cell-specific methylation profile at key genes) as a surrogate for beta cell death in a cohort of autoantibody-positive individuals ( n =32), individuals with very recent-onset type 1 diabetes (<4 months n =92), individuals with long-standing type 1 diabetes (>4 months, n =38) and normoglycaemic/healthy control donors. The authors surprisingly found no significant differences in circulating beta cell DNA in the autoantibody-positive donors vs donors with type 1 diabetes relative to controls [ 52 ]. Despite some limitations with the sensitivity of the assay and the cross-sectional nature of the study, a significant difference in cell-free beta cell DNA was found in donors who had received an islet transplant compared with healthy donors, likely reflecting the extensive beta cell death that frequently occurs post transplant [ 52 ]. Thus, whatever conclusions can be drawn from this study concerning the mode or kinetics of beta cell loss during type 1 diabetes (whether it is progressive, relapsing–remitting, etc.), beta cell death during the early and symptomatic stages of type 1 diabetes is unlikely to release large quantities of cell-free DNA or antigens, as occurs during islet transplantation. It therefore remains to be determined how the mode and kinetics of beta cell death affect the evolution of beta cell antigenicity and neoantigen formation during the development of type 1 diabetes.
Lessons from mouse models
The view that antigen presentation by beta cells is critical for progression of islet autoimmunity has been supported by mechanistic studies manipulating genes in beta cells in the NOD mouse model. Conditional knockout of the unfolded protein response (UPR) mediator Ire1α (also known as Ern1 ) in young beta cells of NOD mice leads to a dedifferentiated phenotype, which alters antigen presentation and halts disease progression [ 53 ]. Adoptive transfer of cytotoxic T cells from mice with beta cell-specific Ire1α deletion failed to induce diabetes in recipient mice [ 53 ], revealing a fundamental phenotypic change in autoimmunity precipitated by the alterations in the beta cells. Similarly, mutation of the type 1 diabetes genome-wide association study gene Rnls , which controls UPR signalling, led to reduced recognition of NIT-1 insulinoma beta cells by polyclonal autoreactive T cells from diabetic NOD mice, without affecting MHC class I or II expression [ 54 ]. Enforced early proliferation of young beta cells in NOD mice by liver insulin receptor knockout (LIRKO) before immune cell infiltration led to profound changes in antigen presentation concomitant with a diminution of autoreactive T cell activity [ 55 ]. NOD mice harbouring Ins1-Cre or a reduced dose of insulin genes show a lower diabetes penetrance but no major changes in autoantibodies or insulitis [ 56 ], and swapping the mouse Ins1 gene for the human INS gene also has a protective effect in NOD mice [ 57 ]. Given the role of insulin as a major autoantigen in type 1 diabetes, the findings from these latter studies are also consistent with altered/reduced presentation of insulin antigens, leading to slower autoimmunity progression and lower type 1 diabetes penetrance in NOD mice. While care should be taken in extrapolating the relevance of results in NOD mice to humans, this work highlights the dynamic relationships between antigen presentation by beta cells and autoimmunity and in particular the significance of beta cell maturation stage.
Beta cell stress responses and islet autoimmunity
A growing number of studies support the view that beta cell stress responses, including the UPR, type I IFN response and senescence, contribute to the progression of islet autoimmunity and type 1 diabetes (reviewed in [ 58 ]). Each of these stress responses occur in human beta cells in type 1 diabetes as shown by immunohistological studies on donor pancreas [ 59 , 60 , 61 ]. Furthermore, drug-based interventions that mitigate each of these stress responses appears to be sufficient to halt diabetes progression in NOD mice (reviewed in [ 62 ]). Moreover, results from clinical trials targeting the UPR in individuals with new-onset type 1 diabetes [ 63 , 64 ] provide support for the translational potential for targeting beta cell stress in humans. Below, we detail each of these stress pathways and what has been learned about their relationships with islet autoimmunity (Fig. 1 ).
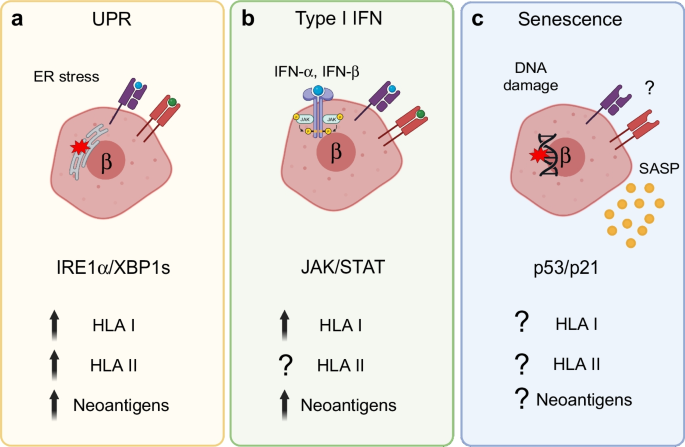
Human beta cell stress responses during the development of type 1 diabetes and effects on antigen presentation. ( a ) ER stress activates the UPR, which either restores homeostasis (adaptive) or initiates apoptosis (terminal). Activation of the UPR may alter antigen presentation via changes in HLA Class I and II expression and formation of neoantigens. ( b ) Type I IFN response is triggered by cytokines IFN-α and IFN-β and leads to activation of JAK/STAT signalling. The IFN response upregulates HLA Class I and II expression and could also lead to the formation of neoantigens via effects on alternative splicing, among other mechanisms. ( c ) Senescence is a stress response characterised by DNA damage, activation of the p53/p21 pathway and an inflammatory secretome (SASP). It is currently unclear how senescence could alter human beta cell antigen presentation but evidence from NOD mice [ 61 ] suggests an impact on Class I and II expression, antigen processing machinery and potential for neoantigen formation. β, beta cell. Created with BioRender.com. This figure is available as part of a downloadable slideset
Beta cell UPR
Beta cells are prone to endoplasmic reticulum (ER) stress as a general consequence of their professional secretory functions [ 65 ]. ER stress activates the UPR, a three-branch signalling cascade that functions to re-establish cellular homeostasis and alleviate ER stress. The three main UPR sensors are inositol requiring enzyme 1 (IRE1), protein kinase R-like endoplasmic reticulum kinase (PERK) and activating transcription factor 6 (ATF6). According to the canonical model of the UPR system in beta cells, if the adaptive UPR fails to reduce chronic ER stress, a terminal UPR occurs, which triggers apoptosis [ 66 ]. Activation of UPR either via chemical stressors or inflammatory cytokines upregulates HLA Class I and II expression, alters antigen presentation by beta cells and elicits neoantigen formation in both mouse and human islets/beta cells [ 45 , 46 , 67 ]. In addition, UPR activation with thapsigargin increases human induced pluripotent stem cell beta cell HLA Class I antigen presentation to type 1 diabetes donor T cells in vitro in an autologous co-culture system [ 68 ]. Most of our knowledge of the UPR in beta cells during type 1 diabetes and its relationship with antigen presentation comes from work on the IRE1α/spliced x-box binding protein 1 (XBP1s) signalling arm [ 66 ] (Fig. 1 a), where changes in this pathway have been linked with altered antigen presentation [ 53 , 67 ]. However, it is not entirely clear whether other UPR mediator(s) also play a role and how sustained the effects on antigen presentation are; the balance between adaptive and terminal UPR may play a significant role.
Beta cell type I IFN response
The type I IFN response is a broadly acting cellular antiviral defence. IFN-α and IFN-β activate janus kinase/signal transducers and activators of transcription (JAK/STAT) pathway signalling leading to a variety of different outcomes, including IFN-stimulated gene expression, ER stress, HLA Class I overexpression and eventual beta cell apoptosis in human donor islets ex vivo and in the human beta cell line EndoC-βH1 [ 69 ]. This stress response also shows a number of genetic associations with type 1 diabetes [ 70 ]. Although upregulation of HLA Class I is observed throughout the islet in type 1 diabetes [ 43 ], other markers of the IFN response occur preferentially in islets with remaining beta cells and insulitis in autoantibody-positive pancreas donors and those with type 1 diabetes [ 71 ]. While treatment with inflammatory cytokines IFN-γ, IL-1β and TNF-α also triggers HLA Class II upregulation in human islet beta cells [ 45 ], whether a similar response occurs following IFN-α or IFN-β stimulation has not been addressed (Fig. 1 b). Interestingly, the RNA editing enzyme adenosine deaminase RNA specific 1 (ADAR1) is upregulated in response to IFN-α but antagonises the IFN pathway by A-to-I editing and alternative splicing of human beta cell mRNAs, thereby altering the transcriptome and potentially leading to neoantigens [ 72 ]. These results highlight the diverse mechanisms by which type I IFN signalling can impact antigen presentation by beta cells and thus could influence progression of islet autoimmunity.
Beta cell senescence
In contrast to both UPR and type I IFN responses both of which can trigger apoptosis, beta cell senescence is a stable anti-apoptotic stress response that involves growth arrest and a proinflammatory secretome termed the senescence-associated secretory phenotype (SASP) [ 62 ]. Single-cell RNA-seq suggested that senescent beta cells in NOD mice upregulate a variety of antigen processing and presentation genes, including MHC I and II [ 61 ], but whether these changes are reflected at the protein level was not determined. Similarly, it has not been determined whether senescence in human islet beta cells elicits changes in HLA Class I or II expression or antigen presentation (Fig. 1 c). Due to SASP and apoptosis resistance, senescent beta cells could persist to continue a long-lasting dialogue with the immune system. Though key details remain to be explored, further investigations into how beta cell senescence alters antigen presentation are clearly warranted.
Do individual beta cells differ in their susceptibility to islet autoimmunity?
Beta cell heterogeneity and islet autoimmunity.
The notion of beta cell functional heterogeneity is increasingly accepted as a natural and biologically relevant feature of islet architecture and health [ 73 , 74 ]. Salomon and Meda first demonstrated the existence of rodent beta cell populations with differing secretory activities [ 75 ] and a few years later, Pipeleers and others [ 76 , 77 ] showed beta cell subpopulations that varied in their metabolic responses to glucose. Transcriptomic and other data (reviewed in [ 78 ]) have now reinforced this view.
An important feature of heterogeneity in islet function is the connectivity between individual beta cells, resulting at least in part from the existence of gap junctional coupling. Supporting the importance of this process, inactivation of the gap junction protein connexin 36 (encoded by Gjd2 ) in beta cells results in defective insulin secretion and glucose tolerance in mice [ 79 , 80 ]. Additionally, highly connected ‘hubs’, representing ~5% of beta cells, that host a disproportionate number of connections to other cells [ 81 ] are involved in the transmission of Ca 2+ signals across the mouse islet. These phenomena are predicted by modelling to play an important role in islet cell physiology [ 82 , 83 ] and appear also to be important in human islets [ 82 ]. Of note, different islets display discrete wave types and behaviours, with ‘hub’ behaviour most prominent in islets displaying more rapid Ca 2+ oscillations [ 84 ]. At least for the functionally defined leader and hub beta cells, respectively, the existence of discrete transcriptomes [ 84 ] and of protein markers including glucokinase [ 81 ] argues that these reflect stable subsets of cells rather than stochastic, functional ‘states’. The relevance of this inter-islet heterogeneity to both normal insulin secretion and type 1 diabetes pathogenesis, is difficult to study given the challenges of interrogating the function of multiple islets while preserving their spatial interrelationships simultaneously within the intact pancreas but may be achievable in pancreatic slice preparations, at least over relatively short ranges (a few mm). Genes involved in modulating beta cell function may also contribute to the hub/leader–follower dynamic. Of particular interest in this context are those genes implicated, through genetics, in the pathogenesis of both type 1 diabetes and type 2 diabetes [ 85 ], such as human GLIS2 and PTPN2 , as well as genes shown in our mouse studies to be enriched ( Gck ) or depleted ( Ins ) in hub cells, or to be enriched (e.g. ADCY6 ) in leader cells [ 84 ]. Single-cell RNA-seq data from individuals with type 1 diabetes and non-diabetic control individuals from the Human Pancreas Analysis Program (HPAP) reveal changes in transcript levels in both beta and alpha cells, with immune-related genes strongly represented in both cell types [ 86 ].
Whether leader or hub (or other) specialised beta cells, or islets enriched in these ‘regulatory’ cell types, are preferentially targeted in type 1 diabetes is not known (Fig. 2 ). Type 1 diabetes-related stressors, such as cytokine treatment, lower the apparent number of hubs in mouse islets [ 87 ] but whether this reflects loss or dysfunction of these cells or weakening of the connections between these and subservient followers is unclear. Likewise, it is unknown whether these specialised beta cell subtypes have any relationship to beta cells that undergo stress responses, such as UPR or senescence, during type 1 diabetes. In any case, preferential loss of leaders or hubs may conceivably lead to islet dysfunction (where a significant number of ‘dormant’ beta cells remain [ 88 ]), in addition to beta cell death, in type 1 diabetes [ 89 ]. Future studies to test this hypothesis will involve quantifying leader and hub cell numbers during the natural history of type 1 diabetes. This may involve live cell analysis in vitro (e.g. using pancreatic slice preparations) or in vivo after engraftment into the anterior eye chamber of immune-compromised mice. An alternative and attractive possibility given their availability (e.g. through the Network for Pancreas Organ Donors with Diabetes, nPOD), is the analysis of fixed samples from individuals with type 1 diabetes. Examination of the mechanisms involved in any selective cell loss, such as preferential expression of conventional or neoantigens, might then be facilitated by prospective studies in vivo [ 90 , 91 ].
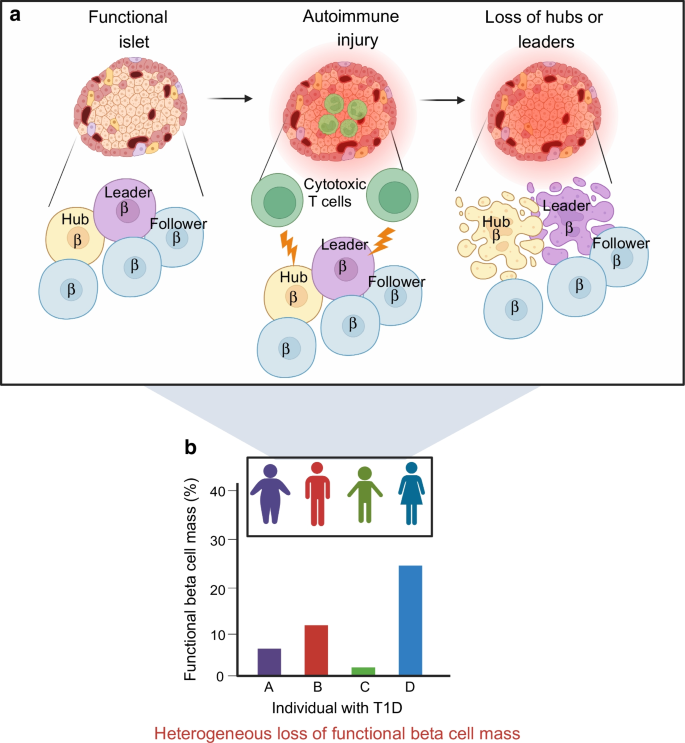
Heterogeneity in the autoimmune response in type 1 diabetes and its potential relationship with inter-individual heterogeneity in residual beta cell mass. ( a ) Islet function is heterogeneous and insulin secretion from the islet is controlled by a small subpopulation of ‘hub’ and ‘leader’ beta cells that coordinate the action of ‘follower’ cells. ( b ) Preferential autoimmune destruction of specialised hub or leader beta cells could help to explain the observation of inter-individual variation in residual functional beta cell mass in individuals with type 1 diabetes. β, beta cell; T1D, type 1 diabetes. Created with BioRender.com. This figure is available as part of a downloadable slideset
Alpha cells and the initiation and progression of islet autoimmunity
Alpha cells in the human islet are much more abundant than in rodents (30–50% of the islet, a value which approaches or may even exceed that of beta cells in some islets) [ 92 ]. During the natural history of type 1 diabetes, alpha cells develop dysfunction including failure of high-glucose-mediated suppression and poor response to low glucose (hypoglycaemic blindness) induced by iatrogenic insulin administration [ 93 ]. It was initially thought that the alpha cell becomes dysregulated only in later stages of type 1 diabetes when loss of beta cell mass (leading to deficient insulin and unrestrained somatostatin secretion) perturbs paracrine modulation of glucagon release (reviewed in [ 94 ]) [ 95 ]. However, reports have shown early loss of the glucagon response to hypoglycaemia in type 1 diabetes [ 96 ]. In fact, the beta cells of islets isolated from single GADA-positive normoglycaemic donors [ 13 ], and those surviving in type 1 diabetes, have remarkably preserved secretory responses [ 97 ]. In contrast, alpha cells from islets isolated from normoglycaemic GADA-positive donors and donors with type 1 diabetes show impaired suppression of glucagon secretion by glucose [ 13 , 97 ]. This would indicate that both intrinsic and extrinsic defects affect the alpha cell in type 1 diabetes.
Alpha cells and the progression of islet autoimmunity
Whereas T cell-mediated destruction of beta cells is well-studied, the collateral damage of this process on alpha cells in the islet microenvironment is poorly understood. A subset of islet-infiltrating T cells recognise peptides derived from proglucagon, and serum autoantibodies to glucagon have been reported in the NOD mouse model [ 98 ], although current evidence has shown this does not occur in humans [ 17 ]. Regardless of the possible mechanisms involved, it is becoming clear that approaches to restore alpha cell function have a beneficial effect on beta cell health and survival in type 1 diabetes. Recent studies targeting glutamate [ 99 ] and glucagon [ 100 ] receptors have demonstrated remarkable efficacy on human type 1 diabetes pancreas slices and type 1 diabetes mouse models in normalising glucagon secretion and improving beta cell mass, respectively. Future studies are required to delineate how aberrant alpha cell function early in type 1 diabetes might influence the progression of the autoimmune attack on beta cells and whether targeting of alpha cell dysfunction could be a viable therapeutic option to ameliorate the progression of islet autoimmunity and/or to preserve beta cell mass and function in humans.
In conclusion, there are many remaining questions surrounding the origins of islet autoimmunity and how it develops during type 1 diabetes. We are only beginning to appreciate the roles of islets and of beta cells themselves in autoimmunity. Early beta cell development as well as the metabolic, phenotypic, transcriptomic and epigenetic reprogramming that beta and alpha cells undergo during diabetogenesis remain poorly understood in humans. Studies within the critical window when seroconversion frequently occurs in infants with high genetic risk will provide key mechanistic insights. We still have much to learn about how beta cell antigen presentation, stress pathways, functional heterogeneity and alpha cells shape the autoimmune response in type 1 diabetes. Sustained progress in this area will reveal the mechanisms underlying heterogeneity in islet autoimmunity and guide our efforts to move clinical interventions in type 1 diabetes towards precision medicine.
Abbreviations
Endoplasmic reticulum
GAD autoantibody
Hybrid insulin peptide
Senescence-associated secretory phenotype
The Environmental Determinants of Diabetes in the Young
Unfolded protein response
Mallone R, Halliez C, Rui J, Herold KC (2022) The β-cell in type 1 diabetes pathogenesis: a victim of circumstances or an instigator of tragic events? Diabetes 71(8):1603–1610. https://doi.org/10.2337/dbi21-0036
Article CAS PubMed PubMed Central Google Scholar
Sims EK, Mirmira RG, Evans-Molina C (2020) The role of beta-cell dysfunction in early type 1 diabetes. Curr Opin Endocrinol Diabetes Obes 27(4):215–224. https://doi.org/10.1097/MED.0000000000000548
Hughes DS, Narendran P (2014) Alpha cell function in type 1 diabetes. Br J Diabetes Vasc Dis 14(2):45–51. https://doi.org/10.15277/bjdvd.2014.014
Article Google Scholar
Herold KC, Bundy BN, Alice Long S et al (2019) An anti-CD3 antibody, teplizumab, in relatives at risk for type 1 diabetes. N Engl J Med 381(7):603–613. https://doi.org/10.1056/NEJMoa1902226
Serra P, Santamaria P (2019) Antigen-specific therapeutic approaches for autoimmunity. Nat Biotechnol 37(3):238–251. https://doi.org/10.1038/s41587-019-0015-4
Article CAS PubMed Google Scholar
Santamaria P (2010) The long and winding road to understanding and conquering type 1 diabetes. Immunity 32(4):437–445. https://doi.org/10.1016/j.immuni.2010.04.003
Tsai S, Shameli A, Santamaria P (2008) CD8+ T cells in type 1 diabetes. Adv Immunol 100:79–124. https://doi.org/10.1016/S0065-2776(08)00804-3
Carré A, Richardson SJ, Larger E, Mallone R (2020) Presumption of guilt for T cells in type 1 diabetes: lead culprits or partners in crime depending on age of onset? Diabetologia 64(1):15–25. https://doi.org/10.1007/s00125-020-05298-y
Battaglia M, Ahmed S, Anderson MS et al (2020) Introducing the endotype concept to address the challenge of disease heterogeneity in type 1 diabetes. Diabetes Care 43(1):5–12. https://doi.org/10.2337/dc19-0880
Article PubMed Google Scholar
Eisenbarth GS (1986) Type I diabetes mellitus. N Engl J Med 314(21):1360–1368. https://doi.org/10.1056/NEJM198605223142106
Insel RA, Dunne JL, Atkinson MA et al (2015) Staging presymptomatic type 1 diabetes: a scientific statement of JDRF, the Endocrine Society, and the American Diabetes Association. Diabetes Care 38(10):1964–1974. https://doi.org/10.2337/dc15-1419
Yu L, Zhao Z, Steck AK (2017) T1D autoantibodies: room for improvement? Curr Opin Endocrinol Diabetes Obes 24(4):285–291. https://doi.org/10.1097/MED.0000000000000348
Doliba NM, Rozo AV, Roman J et al (2022) α Cell dysfunction in islets from nondiabetic, glutamic acid decarboxylase autoantibody–positive individuals. J Clin Invest 132(11):e156243. https://doi.org/10.1172/JCI156243
Vehik K, Bonifacio E, Lernmark Å et al (2020) Hierarchical order of distinct autoantibody spreading and progression to type 1 diabetes in the Teddy study. Diabetes Care 43(9):2066–2073. https://doi.org/10.2337/dc19-2547
Bingley PJ, Boulware DC, Krischer JP (2016) The implications of autoantibodies to a single islet antigen in relatives with normal glucose tolerance: development of other autoantibodies and progression to type 1 diabetes. Diabetologia 59(3):542–549. https://doi.org/10.1007/s00125-015-3830-2
Jacobsen LM, Bocchino L, Evans-Molina C et al (2020) The risk of progression to type 1 diabetes is highly variable in individuals with multiple autoantibodies following screening. Diabetologia 63(3):588–596. https://doi.org/10.1007/s00125-019-05047-w
Anderson AM, Landry LG, Alkanani AA et al (2021) Human islet T cells are highly reactive to preproinsulin in type 1 diabetes. Proc Natl Acad Sci U S A 118(41):e2107208118. https://doi.org/10.1073/pnas.2107208118
Nakayama M, Abiru N, Moriyama H et al (2005) Prime role for an insulin epitope in the development of type 1 diabetes in NOD mice. Nature 435(7039):220–223. https://doi.org/10.1038/nature03523
Delong T, Wiles TA, Baker RL et al (2016) Pathogenic CD4 T cells in type 1 diabetes recognize epitopes formed by peptide fusion. Science 351(6274):711–714. https://doi.org/10.1126/science.aad2791
Wan X, Zinselmeyer BH, Zakharov PN et al (2018) Pancreatic islets communicate with lymphoid tissues via exocytosis of insulin peptides. Nature 560(7716):107–111. https://doi.org/10.1038/s41586-018-0341-6
Umeshappa CS, Mbongue J, Singha S et al (2020) Ubiquitous antigen-specific T regulatory type 1 cells variably suppress hepatic and extrahepatic autoimmunity. J Clin Invest 130(4):1823–1829. https://doi.org/10.1172/JCI130670
Culina S, Lalanne AI, Afonso G et al (2018) Islet-reactive CD8+ T cell frequencies in the pancreas, but not in blood, distinguish type 1 diabetic patients from healthy donors. Sci Immunol 3(20):1–16. https://doi.org/10.1126/sciimmunol.aao4013
Coppieters KT, Dotta F, Amirian N et al (2012) Demonstration of islet-autoreactive CD8 T cells in insulitic lesions from recent onset and long-term type 1 diabetes patients. J Exp Med 209(1):51–60. https://doi.org/10.1084/jem.20111187
Bender C, Rodriguez-Calvo T, Amirian N, Coppieters KT, von Herrath MG (2020) The healthy exocrine pancreas contains preproinsulin-specific CD8 T cells that attack islets in type 1 diabetes. Sci Adv 6(42):1–10. https://doi.org/10.1126/sciadv.abc5586
Article CAS Google Scholar
Yeo L, Pujol-Autonell I, Baptista R et al (2020) Circulating β cell-specific CD8+ T cells restricted by high-risk HLA class I molecules show antigen experience in children with and at risk of type 1 diabetes. Clin Exp Immunol 199(3):263–277. https://doi.org/10.1111/cei.13391
Bender C, Rajendran S, von Herrath MG (2021) New insights into the role of autoreactive CD8 T cells and cytokines in human type 1 diabetes. Front Endocrinol (Lausanne) 11(January):1–6. https://doi.org/10.3389/fendo.2020.606434
Norris JM, Johnson RK, Stene LC (2020) Type 1 diabetes—early life origins and changing epidemiology. Lancet Diabetes Endocrinol 8(3):226–238. https://doi.org/10.1016/S2213-8587(19)30412-7
Rodriguez-Calvo T, Johnson JD, Overbergh L, Dunne JL (2021) Neoepitopes in type 1 diabetes: etiological insights, biomarkers and therapeutic targets. Front Immunol 12(April):1–10. https://doi.org/10.3389/fimmu.2021.667989
Ziegler AG, Bonifacio E (2012) Age-related islet autoantibody incidence in offspring of patients with type 1 diabetes. Diabetologia 55(7):1937–1943. https://doi.org/10.1007/s00125-012-2472-x
Ziegler AG, Rewers M, Simell O et al (2013) Seroconversion to multiple islet autoantibodies and risk of progression to diabetes in children. JAMA 309(23):2473–2479. https://doi.org/10.1001/jama.2013.6285
Vehik K, Lynch KF, Wong MC et al (2019) Prospective virome analyses in young children at increased genetic risk for type 1 diabetes. Nat Med 25(12):1865–1872. https://doi.org/10.1038/s41591-019-0667-0
Krogvold L, Genoni A, Puggioni A et al (2022) Live enteroviruses, but not other viruses, detected in human pancreas at the onset of type 1 diabetes in the DiViD study. Diabetologia 65(12):2108–2120. https://doi.org/10.1007/s00125-022-05779-2
Nekoua MP, Alidjinou EK, Hober D (2022) Persistent coxsackievirus B infection and pathogenesis of type 1 diabetes mellitus. Nat Rev Endocrinol 18(8):503–516. https://doi.org/10.1038/s41574-022-00688-1
Johnson SB, Lynch KF, Roth R et al (2021) First-appearing islet autoantibodies for type 1 diabetes in young children: maternal life events during pregnancy and the child’s genetic risk. Diabetologia 64(3):591–602. https://doi.org/10.1007/s00125-020-05344-9
Warncke K, Weiss A, Achenbach P et al (2022) Elevations in blood glucose before and after the appearance of islet autoantibodies in children. J Clin Invest 132(20):e162123. https://doi.org/10.1172/JCI16212310.1172/JCI162123
Ziegler AG, Achenbach P, Berner R et al (2019) Oral insulin therapy for primary prevention of type 1 diabetes in infants with high genetic risk: the GPPAD-POInT (global platform for the prevention of autoimmune diabetes primary oral insulin trial) study protocol. BMJ Open 9(6):1–9. https://doi.org/10.1136/bmjopen-2018-028578
Abderrahmani A, Jacovetti C, Regazzi R (2022) Lessons from neonatal β-cell epigenomic for diabetes prevention and treatment. Trends Endocrinol Metab 33(6):378–389. https://doi.org/10.1016/j.tem.2022.03.002
Kassem SA, Ariel I, Thornton PS, Scheimberg I, Glaser B (2000) β-Cell proliferation and apoptosis in the developing normal human pancreas and in hyperinsulinism of infancy. Diabetes 49(8):1325–1333. https://doi.org/10.2337/diabetes.49.8.1325
Meier JJ, Köhler CU, Alkhatib B et al (2010) Β-cell development and turnover during prenatal life in humans. Eur J Endocrinol 162(3):559–568. https://doi.org/10.1530/EJE-09-1053
Köhler CU, Olewinski M, Tannapfel A, Schmidt WE, Fritsch H, Meier JJ (2011) Cell cycle control of β-cell replication in the prenatal and postnatal human pancreas. Am J Physiol Endocrinol Metab 300(1):E221-30. https://doi.org/10.1152/ajpendo.00496.2010
Claessens LA, Wesselius J, van Lummel M et al (2020) Clinical and genetic correlates of islet-autoimmune signatures in juvenile-onset type 1 diabetes. Diabetologia 63(2):351–361. https://doi.org/10.1007/s00125-019-05032-3
Li Y, Sun F, Yue T-T et al (2021) Revisiting the antigen-presenting function of β cells in T1D pathogenesis. Front Immunol 12(July):1–8. https://doi.org/10.3389/fimmu.2021.690783
Richardson SJ, Rodriguez-Calvo T, Gerling IC et al (2016) Islet cell hyperexpression of HLA class I antigens: a defining feature in type 1 diabetes. Diabetologia 59(11):2448–2458. https://doi.org/10.1007/s00125-016-4067-4
Russell MA, Redick SD, Blodgett DM et al (2019) HLA class II antigen processing and presentation pathway components demonstrated by transcriptome and protein analyses of islet β-cells from donors with type 1 diabetes. Diabetes 68(5):988–1001. https://doi.org/10.2337/db18-0686
Quesada-Masachs E, Zilberman S, Rajendran S et al (2022) Upregulation of HLA class II in pancreatic beta cells from organ donors with type 1 diabetes. Diabetologia 65(2):387–401. https://doi.org/10.1007/s00125-021-05619-9
Gonzalez-Duque S, Azoury ME, Colli ML et al (2018) Conventional and neo-antigenic peptides presented by β cells are targeted by circulating naïve CD8+ T cells in type 1 diabetic and healthy donors. Cell Metab 28(6):946-960.e6. https://doi.org/10.1016/j.cmet.2018.07.007
Azoury ME, Tarayrah M, Afonso G et al (2020) Peptides derived from insulin granule proteins are targeted by CD8+ T cells across MHC class I restrictions in humans and NOD mice. Diabetes 69(12):2678–2690. https://doi.org/10.2337/db20-0013
Arribas-Layton D, Guyer P, Delong T et al (2020) Hybrid insulin peptides are recognized by human T cells in the context of DRB1*04:01. Diabetes 69(7):1492–1502. https://doi.org/10.2337/db19-0620
Guyer P, Arribas-Layton D, Manganaro A et al (2023) Recognition of mRNA splice variant and secretory granule epitopes by CD4+ T cells in type 1 diabetes. Diabetes 72(1):85–96. https://doi.org/10.2337/db22-0191
Rodriguez-Calvo T, Krogvold L, Amirian N, Dahl-Jørgensen K, von Herrath M (2021) One in ten CD81 cells in the pancreas of living individuals with recent-onset type 1 diabetes recognizes the preproinsulin epitope PPI15-24. Diabetes 70(3):752–758. https://doi.org/10.2337/DB20-0908
Piganelli JD, Mamula MJ, James EA (2021) The role of β cell stress and neo-epitopes in the immunopathology of type 1 diabetes. Front Endocrinol (Lausanne) 11(February):1–10. https://doi.org/10.3389/fendo.2020.624590
Neiman D, Gillis D, Piyanzin S et al (2020) Multiplexing DNA methylation markers to detect circulating cell-free DNA derived from human pancreatic β cells. JCI Insight 5(14):e136579. https://doi.org/10.1172/JCI.INSIGHT.136579
Article PubMed PubMed Central Google Scholar
Lee H, Lee YS, Harenda Q et al (2020) Beta cell dedifferentiation induced by IRE1α deletion prevents type 1 diabetes. Cell Metab 31(4):822-836.e5. https://doi.org/10.1016/j.cmet.2020.03.002
Cai EP, Ishikawa Y, Zhang W et al (2020) Genome-scale in vivo CRISPR screen identifies RNLS as a target for beta cell protection in type 1 diabetes. Nat Metab 2(9):934–945. https://doi.org/10.1038/s42255-020-0254-1
Dirice E, Kahraman S, De Jesus DF et al (2019) Increased β-cell proliferation before immune cell invasion prevents progression of type 1 diabetes. Nat Metab 1(5):509–518. https://doi.org/10.1038/s42255-019-0061-8
Skovsø S, Overby P, Memar-Zadeh J et al (2022) β-cell Cre expression and reduced Ins1 gene dosage protect mice from type 1 diabetes. Endocrinol (United States) 163(11):1–12. https://doi.org/10.1210/endocr/bqac144
Elso CM, Scott NA, Mariana L et al (2019) Replacing murine insulin 1 with human insulin protects NOD mice from diabetes. PLoS One 14(12):1–13. https://doi.org/10.1371/journal.pone.0225021
Roep BO, Thomaidou S, van Tienhoven R, Zaldumbide A (2020) Type 1 diabetes mellitus as a disease of the β-cell (do not blame the immune system?). Nat Rev Endocrinol 17(3):150–161. https://doi.org/10.1038/s41574-020-00443-4
Marhfour I, Lopez XM, Lefkaditis D et al (2012) Expression of endoplasmic reticulum stress markers in the islets of patients with type 1 diabetes. Diabetologia 55(9):2417–2420. https://doi.org/10.1007/s00125-012-2604-3
Marroqui L, Dos Santos RS, Op de beeck A et al (2017) Interferon-α mediates human beta cell HLA class I overexpression, endoplasmic reticulum stress and apoptosis, three hallmarks of early human type 1 diabetes. Diabetologia 60(4):656–667. https://doi.org/10.1007/s00125-016-4201-3
Thompson PJ, Shah A, Ntranos V, Van Gool F, Atkinson M, Bhushan A (2019) Targeted elimination of senescent beta cells prevents type 1 diabetes. Cell Metab 29(5):1045–60. https://doi.org/10.1016/j.cmet.2019.01.021
Brawerman G, Thompson PJ (2020) Beta cell therapies for preventing type 1 diabetes: from bench to bedside. Biomolecules 10(12):1–20. https://doi.org/10.3390/biom10121681
Ovalle F, Grimes T, Xu G et al (2018) Verapamil and beta cell function in adults with recent-onset type 1 diabetes. Nat Med 24(8):1108–1112. https://doi.org/10.1038/s41591-018-0089-4
Gitelman SE, Bundy BN, Ferrannini E et al (2021) Imatinib therapy for patients with recent-onset type 1 diabetes: a multicentre, randomised, double-blind, placebo-controlled, phase 2 trial. Lancet Diabetes Endocrinol 9(8):502–514. https://doi.org/10.1016/S2213-8587(21)00139-X
Mallone R, Eizirik DL (2020) Presumption of innocence for beta cells: why are they vulnerable autoimmune targets in type 1 diabetes? Diabetologia 63(10):1999–2006. https://doi.org/10.1007/s00125-020-05176-7
Sahin GS, Lee H, Engin F (2021) An accomplice more than a mere victim: the impact of β-cell ER stress on type 1 diabetes pathogenesis. Mol Metab 54:101365. https://doi.org/10.1016/j.molmet.2021.101365
Thomaidou S, Kracht MJL, Van Der Slik A et al (2020) β-cell stress shapes CTL immune recognition of preproinsulin signal peptide by posttranscriptional regulation of endoplasmic reticulum aminopeptidase 1. Diabetes 69(4):670–680. https://doi.org/10.2337/db19-0984
Leite NC, Sintov E, Meissner TB et al (2020) Modeling type 1 diabetes in vitro using human pluripotent stem cells. Cell Rep 32(2):107894. https://doi.org/10.1016/j.celrep.2020.107894
Marroqui L, Perez-Serna AA, Babiloni-Chust I, Dos Santos RS (2021) Type I interferons as key players in pancreatic β-cell dysfunction in type 1 diabetes. Int Rev Cell Mol Biol 359:1–80. https://doi.org/10.1016/BS.IRCMB.2021.02.011
Størling J, Pociot F (2017) Type 1 diabetes candidate genes linked to pancreatic islet cell inflammation and beta-cell apoptosis. Genes (Basel) 8(2):1–12. https://doi.org/10.3390/genes8020072
Apaolaza PS, Balcacean D, Zapardiel-Gonzalo J et al (2021) Islet expression of type I interferon response sensors is associated with immune infiltration and viral infection in type 1 diabetes. Sci Adv 7(9):eabd6527. https://doi.org/10.1126/sciadv.abd6527
Szymczak F, Cohen-Fultheim R, Thomaidou S et al (2022) ADAR1-dependent editing regulates human β cell transcriptome diversity during inflammation. Front Endocrinol (Lausanne) 13(November):1–12. https://doi.org/10.3389/fendo.2022.1058345
Miranda MA, Macias-Velasco JF, Lawson HA (2021) Pancreatic β-cell heterogeneity in health and diabetes: classes, sources, and subtypes. Am J Physiol Endocrinol Metab 320(4):E716–E731. https://doi.org/10.1152/AJPENDO.00649.2020
Chabosseau P, Rutter GA, Millership SJ (2021) Importance of both imprinted genes and functional heterogeneity in pancreatic beta cells: is there a link? Int J Mol Sci 22(3):1000. https://doi.org/10.3390/ijms22031000
Salomon D, Meda P (1986) Heterogeneity and contact-dependent regulation of hormone secretion by individual B cells. Exp Cell Res 162(2):507–520. https://doi.org/10.1016/0014-4827(86)90354-x
Pipeleers DG (1992) Heterogeneity in pancreatic beta-cell population. Diabetes 41(7):777–781. https://doi.org/10.2337/diab.41.7.777
Van Schravendijk CF, Kiekens R, Pipeleers DG (1992) Pancreatic beta cell heterogeneity in glucose-induced insulin secretion. J Biol Chem 267(30):21344–21348. https://doi.org/10.1016/S0021-9258(19)36615-3
Gutierrez GD, Gromada J, Sussel L (2017) Heterogeneity of the pancreatic beta cell. Front Genet 8(MAR):1–9. https://doi.org/10.3389/fgene.2017.00022
Ravier MA, Güldenagel M, Charollais A et al (2005) Loss of connexin36 channels alters beta-cell coupling, islet synchronization of glucose-induced Ca2+ and insulin oscillations, and basal insulin release. Diabetes 54(6):1798–1807. https://doi.org/10.2337/diabetes.54.6.1798
Benninger RKP, Zhang M, Head WS, Satin LS, Piston DW (2008) Gap junction coupling and calcium waves in the pancreatic islet. Biophys J 95(11):5048–5061. https://doi.org/10.1529/biophysj.108.140863
Johnston NR, Mitchell RK, Haythorne E et al (2016) Beta cell hubs dictate pancreatic islet responses to glucose. Cell Metab 24(3):389–401. https://doi.org/10.1016/j.cmet.2016.06.020
Gosak M, Stožer A, Markovič R et al (2017) Critical and supercritical spatiotemporal calcium dynamics in beta cells. Front Physiol 8:1106. https://doi.org/10.3389/fphys.2017.01106
Dwulet JM, Briggs JK, Benninger RKP (2021) Small subpopulations of β-cells do not drive islet oscillatory [Ca2+] dynamics via gap junction communication. PLoS Comput Biol 17(5):e1008948. https://doi.org/10.1371/journal.pcbi.1008948
Chasbosseau P, Yong F, Delgadillo-Silva L et al (2023) Molecular phenotyping of single pancreatic islet leader beta cells by Flash-Seq. Life Sci 316(October 2022):121436. https://doi.org/10.1016/j.lfs.2023.121436
Inshaw JRJ, Sidore C, Cucca F et al (2021) Analysis of overlapping genetic association in type 1 and type 2 diabetes. Diabetologia 64(6):1342–1347. https://doi.org/10.1007/s00125-021-05428-0
Bosi E, Marchetti P, Rutter GA, Eizirik DL (2022) Human alpha cell transcriptomic signatures of types 1 and 2 diabetes highlight disease-specific dysfunction pathways. iScience 25(10):105056. https://doi.org/10.1016/j.isci.2022.105056
Hodson DJ, Mitchell RK, Bellomo EA et al (2013) Lipotoxicity disrupts incretin-regulated human β cell connectivity. J Clin Invest 123(10):4182–4194. https://doi.org/10.1172/JCI68459
Oram RA, McDonald TJ, Shields BM et al (2015) Most people with long-duration type 1 diabetes in a large population-based study are insulin microsecretors. Diabetes Care 38(2):323–328. https://doi.org/10.2337/dc14-0871
Benninger RKP, Dorrell C, Hodson DJ, Rutter GA (2018) The impact of pancreatic beta cell heterogeneity on type 1 diabetes pathogenesis. Curr Diab Rep 18(11):112. https://doi.org/10.1007/s11892-018-1085-2
Abdulreda MH, Molano RD, Faleo G et al (2019) In vivo imaging of type 1 diabetes immunopathology using eye-transplanted islets in NOD mice. Diabetologia 62(7):1237–1250. https://doi.org/10.1007/s00125-019-4879-0
Salem V, Silva LD, Suba K et al (2019) Leader β-cells coordinate Ca(2+) dynamics across pancreatic islets in vivo. Nat Metab 1(6):615–629. https://doi.org/10.1038/s42255-019-0075-2
Cabrera O, Berman DM, Kenyon NS, Ricordi C, Berggren PO, Caicedo A (2006) The unique cytoarchitecture of human pancreatic islets has implications for islet cell function. Proc Natl Acad Sci U S A 103(7):2334–2339. https://doi.org/10.1073/pnas.0510790103
Cryer PE (2012) Minireview: glucagon in the pathogenesis of hypoglycemia and hyperglycemia in diabetes. Endocrinology 153(3):1039–1048. https://doi.org/10.1210/en.2011-1499
Gaisano HY, MacDonald PE, Vranic M (2012) Glucagon secretion and signaling in the development of diabetes. Front Physiol 3(September):1–12. https://doi.org/10.3389/fphys.2012.00349
Karimian N, Qin T, Liang T et al (2013) Somatostatin receptor type 2 antagonism improves glucagon counterregulation in biobreeding diabetic rats. Diabetes 62(8):2968–2977. https://doi.org/10.2337/db13-0164
Siafarikas A, Johnston RJ, Bulsara MK, O’Leary P, Jones TW, Davis EA (2012) Early loss of the glucagon response to hypoglycemia in adolescents with type 1 diabetes. Diabetes Care 35(8):1757–1762. https://doi.org/10.2337/dc11-2010
Brissova M, Haliyur R, Saunders D et al (2018) α cell function and gene expression are compromised in type 1 diabetes. Cell Rep 22(10):2667–2676. https://doi.org/10.1016/j.celrep.2018.02.032
Mukherjee G, Chaparro RJ, Schloss J, Smith C, Bando CD, Dilorenzo TP (2015) Glucagon-reactive islet-infiltrating CD8 T cells in NOD mice. Immunology 144(4):631–640. https://doi.org/10.1111/imm.12415
Panzer JK, Tamayo A, Caicedo A (2022) Restoring glutamate receptor signaling in pancreatic alpha cells rescues glucagon responses in type 1 diabetes. Cell Rep 41(11):111792. https://doi.org/10.1016/j.celrep.2022.111792
Wang MY, Dean ED, Quittner-Strom E et al (2021) Glucagon blockade restores functional β-cell mass in type 1 diabetic mice and enhances function of human islets. Proc Natl Acad Sci U S A 118(9):1–8. https://doi.org/10.1073/pnas.2022142118
Download references
Author information
Authors and affiliations.
Children’s Hospital Research Institute of Manitoba, Winnipeg, MB, Canada
Peter J. Thompson & Jasmine Pipella
Department of Physiology & Pathophysiology, University of Manitoba, Winnipeg, MB, Canada
CRCHUM and Department of Medicine, Université de Montréal, Montréal, QC, Canada
Guy A. Rutter
Department of Diabetes, Endocrinology and Medicine, Faculty of Medicine, Imperial College, London, UK
LKC School of Medicine, Nanyang Technological College, Singapore, Republic of Singapore
Departments of Medicine and Physiology, University of Toronto, Toronto, ON, Canada
Herbert Y. Gaisano
Cumming School of Medicine, University of Calgary, Calgary, AB, Canada
Pere Santamaria
Institut D’Investigacions Biomèdiques August Pi i Sunyer (IDIBAPS), Barcelona, Spain
You can also search for this author in PubMed Google Scholar
Corresponding authors
Correspondence to Peter J. Thompson or Guy A. Rutter .
Ethics declarations
Acknowledgements.
We apologise to colleagues whose work could not be cited due to space limitations.
PJT was supported by CIHR (PJT-479641), a new investigator operating grant from Research Manitoba (5351), an operating grant from the Health Sciences Centre Foundation Winnipeg and an End Diabetes 2022 Award from Diabetes Canada (OG-3-22-5694-PT). GAR was supported by a Wellcome Trust Investigator Award (212625/Z/18/Z), UK MRC Programme grant (MR/R022259/1), Diabetes UK Project grant (BDA16/0005485), CRCHUM start-up funds, an Innovation Canada John R. Evans Leader Award (CFI 42649) and NIH-NIDDK (R01DK135268) project grant. HYG was supported by CIHR (PJT-159741 and PJT-148652) and the Canada Research Chairs Program. PS was supported by CIHR (FDN-353029, PJT-479040, PJT-479038, FRN-168480 [with JDRF], DT4-179512), Genome Canada (GAPP program), the Praespero Foundation, the Alberta Diabetes Foundation, the ISCIII and FEDER (PIE14/00027, PI15/0797), MINECO (PID2021-125493OB-I00), Generalitat de Catalunya (SGR and CERCA Programmes) and Red Española de Supercomputación (RES, providing CSUC resources). All investigators were supported by a CIHR-JDRF team grant (CIHR-IRSC TDP-186358 and JDRF 4-SRA-2023-1182-S-N).
Authors’ relationships and activities
GAR has received grant funding from, and is a consultant for, Sun Pharmaceuticals Inc. PS is founder, scientific officer and stockholder of Parvus Therapeutics. He is inventor on patents on pMHC-based nanomedicines and receives funding from the company. The other authors declare that there are no relationships or activities that might bias, or be perceived to bias, their work.
Contribution statement
All authors conceived of the manuscript and provided contributions. PJT and PS wrote the sections on islet autoimmunity initiation, antigen presentation and early life exposures. JP wrote the section on beta cell stress pathways with guidance from PJT. GAR wrote the section on beta cell functional heterogeneity. HYG wrote the section on alpha cell dysfunction. All authors edited and approved the final version of the manuscript.
Additional information
Publisher's note.
Springer Nature remains neutral with regard to jurisdictional claims in published maps and institutional affiliations.
Supplementary Information
Below is the link to the electronic supplementary material.
Supplementary file1 (PPTX 506 KB)
Rights and permissions.
Open Access This article is licensed under a Creative Commons Attribution 4.0 International License, which permits use, sharing, adaptation, distribution and reproduction in any medium or format, as long as you give appropriate credit to the original author(s) and the source, provide a link to the Creative Commons licence, and indicate if changes were made. The images or other third party material in this article are included in the article's Creative Commons licence, unless indicated otherwise in a credit line to the material. If material is not included in the article's Creative Commons licence and your intended use is not permitted by statutory regulation or exceeds the permitted use, you will need to obtain permission directly from the copyright holder. To view a copy of this licence, visit http://creativecommons.org/licenses/by/4.0/ .
Reprints and permissions
About this article
Thompson, P.J., Pipella, J., Rutter, G.A. et al. Islet autoimmunity in human type 1 diabetes: initiation and progression from the perspective of the beta cell. Diabetologia 66 , 1971–1982 (2023). https://doi.org/10.1007/s00125-023-05970-z
Download citation
Received : 10 March 2023
Accepted : 30 May 2023
Published : 25 July 2023
Issue Date : November 2023
DOI : https://doi.org/10.1007/s00125-023-05970-z
Share this article
Anyone you share the following link with will be able to read this content:
Sorry, a shareable link is not currently available for this article.
Provided by the Springer Nature SharedIt content-sharing initiative
- Alpha cells
- Islet autoimmunity
- Type 1 diabetes
- Find a journal
- Publish with us
- Track your research
Novel Stem Cell-Derived Islet Cell Therapy Continues to Show Promise for Achieving Insulin Independence for Individuals with Type 1 Diabetes
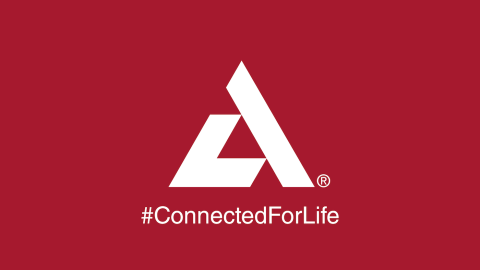
Stem Cell-Derived Fully Differentiated Islet Cells Shown to Restore Endogenous Insulin Secretion and Improved Glycemic Control in People Living with Type 1 Diabetes Today, findings from the ongoing clinical trial of VX-880, a phase 1/2, multi-center, single-arm, open-label study in patients with type 1 diabetes (T1D) were presented, demonstrating the potential of stem cell-derived islet cell therapy as a future treatment option for patients with T1D. All patients treated with VX-880 had undetectable insulin secretion and a history of recurrent severe hypoglycemic events (SHE) in the year prior to treatment; following treatment, all six patients demonstrated restored insulin secretion, improved glycemic control, improved time-in-range, reduction or elimination of exogenous insulin usage and complete absence of SHEs in the post-Day 90 evaluation period. The results were presented at the 83 rd Scientific Sessions of the American Diabetes Association ® (ADA) in San Diego, CA. The study is focused on adult T1D patients with impaired hypoglycemic awareness and severe hypoglycemia. Hypoglycemia, known as low blood glucose, is common in individuals with T1D and often occurs because of imbalances between insulin administered relative to an individual’s requirements at a particular time that can be affected by a multitude of factors e.g., diet, sleep, stress, activity levels. 1 Over time, people with T1D may lose awareness of hypoglycemia, meaning they may not feel symptoms despite blood glucose readings falling below a level which normally may provoke symptoms, usually below 70 mg/dL. If left untreated, this can lead to severe hypoglycemic events, which can present with loss of consciousness, coma, seizures or serious injury as such, these events can potentially be fatal. Current standards of care do not address the underlying causes of the disease, and there are limited treatment options beyond exogenous insulin for the management of T1D. Two patients treated with VX-880 (one in Part A of the study who, per protocol, received half the target dose, and one in Part B who received the full target dose) were treated for at least 12 months of follow-up and were evaluable for the study’s primary efficacy endpoint of elimination of SHEs between Day 90 and Month 12 with HbA1c of <7%. Both patients treated for more than 12 months are insulin independent; Patient A1 had HbA1c of 5.3% at Month 21 (compared to 8.6% at baseline) Patient B1 had HbA1c had HbA1c of 6.0% at Month 12 (compared to 7.6% at baseline). This level of glucose control is highly unusual in T1D patients treated with exogenous insulin, with recent data indicating that only approximately 25% of people with T1D meet the recommended HbA1c target of 7.0%. In fact, both patients displayed HbA1c levels that are below the diagnostic threshold for diabetes (6.5%). Both patients also showed over 95% time-in-range, well above the ADA recommended target of 70%, and substantial improvement over baseline. The three additional patients in Part B, each administered the full target dose of VX-880 given as a single infusion, have had up to 90 days of follow-up and also have shown insulin production, reduction in HbA1c, improvements in time-in-range and reductions in daily insulin usage. Their trajectory is consistent with that observed in the two patients with more than one year of follow up at equivalent periods of follow-up after VX-880 infusion. VX-880 has been generally safe and well tolerated in all patients dosed to date, with a safety profile consistent with immunosuppressant therapy and cadaveric islet cell transplantation. The majority of adverse events (AEs) were mild or moderate and there were no SAEs related to VX-880 treatment. “These new findings demonstrate the potential of stem cell-derived islets as a future treatment for patients with type 1 diabetes, signaling a new era that could potentially remove the need for exogenously administered insulin to achieve glycemic control,” said Trevor W. Reichman, MD, PhD, Surgical Director of Pancreas and Islet Cell Transplantation, Ajmera Transplant Center at the University of Toronto. “We are hopeful that this first-of-its-kind research could be a gamechanger for the treatment of type 1 diabetes.” As a result of these positive safety and efficacy data in Parts A and B, an independent data review committee has recommended moving to Part C of the trial, which allows for concurrent dosing of patients at the full target dose of VX-880. The VX-880 trial has expanded to additional sites that are active and enrolling in Norway, Switzerland and the Netherlands. Research presentation details: Dr. Reichman presented the findings at the following sessions:
- Glucose-Dependent Insulin Production and Insulin-Independence in Type 1 Diabetes from Stem Cell–Derived, Fully Differentiated Islet Cells—Updated Data from the VX-880 Clinical Trial
- Presented on Friday, June 23, 2023 in San Diego, California at 3:50pm PT as an oral presentation. These data will also be presented as a poster presentation on Sunday, June 25 at 11:30am PT (poster #836-P)
About the ADA’s Scientific Sessions The ADA's 83rd Scientific Sessions, the world's largest scientific meeting focused on diabetes research, prevention, and care, will be held in San Diego, CA on June 23–26. More than 12,000 leading physicians, scientists, and health care professionals from around the world are expected to convene both in person and virtually to unveil cutting-edge research, treatment recommendations, and advances toward a cure for diabetes. Attendees will receive exclusive access to thousands of original research presentations and take part in provocative and engaging exchanges with leading diabetes experts. Join the Scientific Sessions conversation on social media using #ADA2023
About the American Diabetes Association The American Diabetes Association (ADA) is the nation’s leading voluntary health organization fighting to bend the curve on the diabetes epidemic and help people living with diabetes thrive. For 82 years, the ADA has driven discovery and research to treat, manage, and prevent diabetes while working relentlessly for a cure. Through advocacy, program development, and education we aim to improve the quality of life for the over 133 million Americans living with diabetes or prediabetes. Diabetes has brought us together. What we do next will make us Connected for Life ® . To learn more or to get involved, visit us at diabetes.org or call 1-800-DIABETES (1-800-342-2383). Join the fight with us on Facebook ( American Diabetes Association ), Spanish Facebook ( Asociación Americana de la Diabetes ), LinkedIn ( American Diabetes Association ), Twitter ( @AmDiabetesAssn ), and Instagram ( @AmDiabetesAssn ).
1 https://diabetes.org/healthy-living/medication-treatments/blood-glucose-testing-and-control/hypoglycemia
Contact Virginia Cramer for press-related questions.
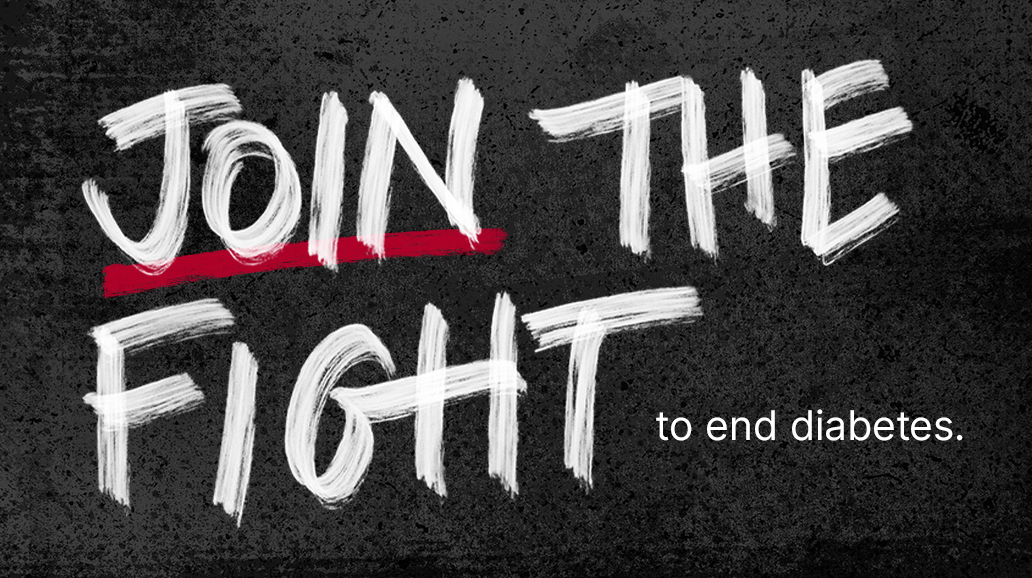
Give Today and Change lives!
With your support, the American Diabetes Association® can continue our lifesaving work to make breakthroughs in research and provide people with the resources they need to fight diabetes.
Thank you for visiting nature.com. You are using a browser version with limited support for CSS. To obtain the best experience, we recommend you use a more up to date browser (or turn off compatibility mode in Internet Explorer). In the meantime, to ensure continued support, we are displaying the site without styles and JavaScript.
- View all journals
- Explore content
- About the journal
- Publish with us
- Sign up for alerts
- Perspective
- Published: 11 August 2022
Every islet matters: improving the impact of human islet research
- Anna L. Gloyn ORCID: orcid.org/0000-0003-1205-1844 1 ,
- Mark Ibberson ORCID: orcid.org/0000-0003-3152-5670 2 ,
- Piero Marchetti 3 ,
- Alvin C. Powers ORCID: orcid.org/0000-0003-1941-5786 4 , 5 ,
- Patrik Rorsman ORCID: orcid.org/0000-0001-7578-0767 6 , 7 ,
- Maike Sander 8 &
- Michele Solimena 9 , 10
Nature Metabolism volume 4 , pages 970–977 ( 2022 ) Cite this article
1964 Accesses
15 Citations
97 Altmetric
Metrics details
- Type 2 diabetes
An Author Correction to this article was published on 02 July 2024
This article has been updated
Detailed characterization of human pancreatic islets is key to elucidating the pathophysiology of all forms of diabetes, especially type 2 diabetes. However, access to human pancreatic islets is limited. Pancreatic tissue for islet retrieval can be obtained from brain-dead organ donors or from individuals undergoing pancreatectomy, often referred to as ‘living donors’. Different protocols for human islet procurement can substantially impact islet function. This variability, coupled with heterogeneity between individuals and islets, results in analytical challenges to separate genuine disease pathology or differences between human donors from experimental noise. There are currently no international guidelines for human donor phenotyping, islet procurement and functional characterization. This lack of standardization means that substantial investments from multiple international efforts towards improved understanding of diabetes pathology cannot be fully leveraged. In this Perspective, we overview the status of the field of human islet research, highlight the challenges and propose actions that could accelerate research progress and increase understanding of type 2 diabetes to slow its pandemic spreading.
This is a preview of subscription content, access via your institution
Access options
Access Nature and 54 other Nature Portfolio journals
Get Nature+, our best-value online-access subscription
24,99 € / 30 days
cancel any time
Subscribe to this journal
Receive 12 digital issues and online access to articles
111,21 € per year
only 9,27 € per issue
Buy this article
- Purchase on SpringerLink
- Instant access to full article PDF
Prices may be subject to local taxes which are calculated during checkout
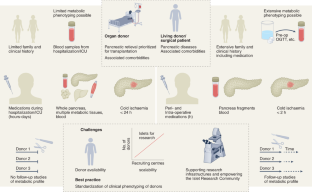
Similar content being viewed by others
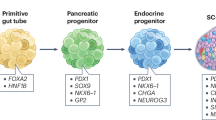
Harnessing cellular therapeutics for type 1 diabetes mellitus: progress, challenges, and the road ahead
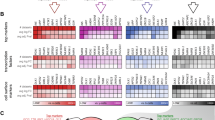
Generation of human islet cell type-specific identity genesets

Single-cell multi-omics analysis of human pancreatic islets reveals novel cellular states in type 1 diabetes
Change history, 02 july 2024.
A Correction to this paper has been published: https://doi.org/10.1038/s42255-024-01091-y
Rickels, M. R. & Robertson, R. P. Pancreatic islet transplantation in humans: recent progress and future directions. Endocr. Rev. 40 , 631–668 (2019).
Article PubMed Google Scholar
Alonso, L. et al. TIGER: the gene expression regulatory variation landscape of human pancreatic islets. Cell Rep. 37 , 109807 (2021).
Article CAS PubMed PubMed Central Google Scholar
Asplund, O. et al. Islet Gene View—a tool to facilitate islet research. Preprint at bioRxiv https://doi.org/10.1101/435743 (2020).
Brissova, M. et al. The Integrated Islet Distribution Program answers the call for improved human islet phenotyping and reporting of human islet characteristics in research articles. Diabetologia 62 , 1312–1314 (2019).
Article PubMed PubMed Central Google Scholar
Kaestner, K. H., Powers, A. C., Naji, A. & Atkinson, M. A. NIH initiative to improve understanding of the pancreas, islet, and autoimmunity in type 1 diabetes: the Human Pancreas Analysis Program (HPAP). Diabetes 68 , 1394–1402 (2019).
Marchetti, P. et al. Fostering improved human islet research: a European perspective. Diabetologia 62 , 1514–1516 (2019).
Solimena, M. et al. Systems biology of the IMIDIA biobank from organ donors and pancreatectomised patients defines a novel transcriptomic signature of islets from individuals with type 2 diabetes. Diabetologia 61 , 641–657 (2018).
Article CAS PubMed Google Scholar
Fadista, J. et al. Global genomic and transcriptomic analysis of human pancreatic islets reveals novel genes influencing glucose metabolism. Proc. Natl Acad. Sci. USA 111 , 13924–13929 (2014).
Rosengren, A. H. et al. Reduced insulin exocytosis in human pancreatic β-cells with gene variants linked to type 2 diabetes. Diabetes 61 , 1726–1733 (2012).
Taneera, J. et al. Identification of novel genes for glucose metabolism based upon expression pattern in human islets and effect on insulin secretion and glycemia. Hum. Mol. Genet. 24 , 1945–1955 (2015).
Taneera, J. et al. A systems genetics approach identifies genes and pathways for type 2 diabetes in human islets. Cell Metab. 16 , 122–134 (2012).
Viñuela, A. et al. Genetic variant effects on gene expression in human pancreatic islets and their implications for T2D. Nat. Commun. 11 , 4912 (2020).
Marselli, L. et al. Persistent or transient human beta cell dysfunction induced by metabolic stress: specific signatures and shared gene expression with type 2 diabetes. Cell Rep. 33 , 108466 (2020).
Wigger, L. et al. Multi-omics profiling of living human pancreatic islet donors reveals heterogeneous beta cell trajectories towards type 2 diabetes. Nat. Metab. 3 , 1017–1031 (2021).
Marchetti, P., Suleiman, M. & Marselli, L. Organ donor pancreases for the study of human islet cell histology and pathophysiology: a precious and valuable resource. Diabetologia 61 , 770–774 (2018).
Krogvold, L. et al. Pancreatic biopsy by minimal tail resection in live adult patients at the onset of type 1 diabetes: experiences from the DiViD study. Diabetologia 57 , 841–843 (2014).
Atkinson, M. A. Pancreatic biopsies in type 1 diabetes: revisiting the myth of Pandora’s box. Diabetologia 57 , 656–659 (2014).
Henquin, J. C. Glucose-induced insulin secretion in isolated human islets: does it truly reflect β-cell function in vivo? Mol. Metab. 48 , 101212 (2021).
Negi, S. et al. Analysis of beta-cell gene expression reveals inflammatory signaling and evidence of dedifferentiation following human islet isolation and culture. PLoS ONE 7 , e30415 (2012).
Mawla, A. M. & Huising, M. O. Navigating the depths and avoiding the shallows of pancreatic islet cell transcriptomes. Diabetes 68 , 1380–1393 (2019).
Barklin, A. Systemic inflammation in the braindead organ donor. Acta Anaesthesiol. Scand. 53 , 425–435 (2009).
Boggi, U. et al. First world consensus conference on pancreas transplantation: part I—methods and results of literature search. Am. J. Transplant. 21 , 1–16 (2021).
Berney, T. et al. Utilization of organs from donors after circulatory death for vascularized pancreas and islet of Langerhans transplantation: recommendations from an expert group. Transpl. Int. 29 , 798–806 (2016).
Markmann, J. F. et al. The use of non-heart-beating donors for isolated pancreatic islet transplantation. Transplantation 75 , 1423–1429 (2003).
Barovic, M. et al. Metabolically phenotyped pancreatectomized patients as living donors for the study of islets in health and diabetes. Mol. Metab. 27S , S1–S6 (2019).
Mezza, T. et al. Increased beta-cell workload modulates proinsulin-to-insulin ratio in humans. Diabetes 67 , 2389–2396 (2018).
Mezza, T. et al. Pancreaticoduodenectomy model demonstrates a fundamental role of dysfunctional beta cells in predicting diabetes. J. Clin. Invest. 131 , e146788 (2021).
Niwano, F. et al. Glucose metabolism after pancreatectomy: opposite extremes between pancreaticoduodenectomy and distal pancreatectomy. J. Clin. Endocrinol. Metab. 106 , e2203–e2214 (2021).
Wang, Y. et al. Paraneoplastic beta cell dedifferentiation in nondiabetic patients with pancreatic cancer. J. Clin. Endocrinol. Metab. 105 , dgz224 (2020).
Panzer, J. K., Cohrs, C. M. & Speier, S. Using pancreas tissue slices for the study of islet physiology. Methods Mol. Biol. 2128 , 301–312 (2020).
Brereton, M. F., Vergari, E., Zhang, Q. & Clark, A. Alpha-, delta- and PP-cells: are they the architectural cornerstones of islet structure and coordination? J. Histochem. Cytochem. 63 , 575–591 (2015).
Ahlqvist, E. et al. Novel subgroups of adult-onset diabetes and their association with outcomes: a data-driven cluster analysis of six variables. Lancet Diabetes Endocrinol. 6 , 361–369 (2018).
Walker, J. T. et al. The human islet: mini-organ with mega impact. Endocr. Rev. 42 , 605–657 (2021).
Manning Fox, J. E. et al. Human islet function following 20 years of cryogenic biobanking. Diabetologia 58 , 1503–1512 (2015).
Henquin, J. C. The challenge of correctly reporting hormones content and secretion in isolated human islets. Mol. Metab. 30 , 230–239 (2019).
Verge, C. F. et al. Combined use of autoantibodies (IA-2 autoantibody, GAD autoantibody, insulin autoantibody, cytoplasmic islet cell antibodies) in type 1 diabetes: combinatorial islet autoantibody workshop. Diabetes 47 , 1857–1866 (1998).
Wilkinson, M. D. et al. The FAIR guiding principles for scientific data management and stewardship. Sci. Data 3 , 160018 (2016).
Dragan, I., Sparsø, T., Kuznetsov, D., Slieker, R. & Ibberson, M. SwissKnife: an R package for federated data analysis. Preprint at bioRxiv https://doi.org/10.1101/2020.11.17.386813 (2020).
Warnat-Herresthal, S. et al. Swarm Learning for decentralized and confidential clinical machine learning. Nature 594 , 265–270 (2021).
Wagner, R. et al. Pathophysiology-based subphenotyping of individuals at elevated risk for type 2 diabetes. Nat. Med. 27 , 49–57 (2021).
Download references
Acknowledgements
We are grateful to all islet donors and their families for their support in this endeavour. We thank F. J. Moller for helping with figure preparation. A.L.G. is a Wellcome Senior Fellow in Basic Biomedical Science. A.L.G. is funded by Wellcome (200837) and NIDDK (U01-DK105535, U01-DK085545, UM1DK126185, U01DK123743 and U24DK098085) and the Stanford Diabetes Research Center (NIDDK award P30DK116074). P.M. is supported by the Italian Ministry of University and Research, PRIN 2017, Molecular and Pathophysiological Heterogeneity of Autoimmune Diabetes: Implication for Precision Medicine, 2017KAM2R5_005. A.C.P. is funded by the HIRN (RRID: SCR_014393 ), the Human Pancreas Analysis Program (RRID: SCR_016202 ), DK106755, DK123716, DK112232, DK112217 and DK20593 (Vanderbilt Diabetes Research and Training Center), The Leona M. and Harry B. Helmsley Charitable Trust, and the Department of Veterans Affairs (BX000666). P.R. is funded by the Medical Research Council, the Swedish Research Council and The Leona M. and Harry B. Helmsley Charitable Trust. M. Sander is supported by grants from the National Institutes of Health (R01DK078803, R01DK068471, R01DK114427, R01DK122607, U01DK120429, U01HG012059 and UH3DK122639). M. Solimena is funded by the BMBF-German Center for Diabetes Research (DZD e.V.), the Deutsche Forschungsgemeinschaft (DFG; grants SO 818/10-1 and IRTG2251) and the DFG-ANR programme (SO 818/6-1). This work is also supported with funds by the Innovative Medicines Initiative 2 Joint Undertaking under grant agreement no. 115881 (RHAPSODY) to M. Solimena, P.M. and M.I., and no. 115797 (INNODIA) and no. 945268 (INNODIA HARVEST) to M. Solimena and P.M. This Joint Undertaking receives support from the EU Horizon 2020 research and innovation programme, EFPIA, JDRF and The Leona M. and Harry B. Helmsley Charitable Trust. This work is further supported by the Swiss State Secretariat for Education‚ Research and Innovation under contract number 16.0097-2. The opinions expressed and arguments used herein do not necessarily reflect the official views of these funding bodies.
Author information
Authors and affiliations.
Department of Pediatrics, Division of Endocrinology & Stanford Diabetes Research Center, Stanford University, Stanford, CA, USA
Anna L. Gloyn
Vital-IT, SIB Swiss Institute of Bioinformatics, Lausanne, Switzerland
Mark Ibberson
Department of Clinical and Experimental Medicine, University of Pisa, Pisa, Italy
Piero Marchetti
Vanderbilt University Medical Center, Nashville, TN, USA
Alvin C. Powers
VA Tennessee Valley Healthcare System, Nashville, TN, USA
Oxford Centre for Diabetes, Endocrinology and Metabolism, Radcliffe Department of Medicine, University of Oxford, Oxford, UK
Patrik Rorsman
Metabolic Physiology Unit, Institute of Neuroscience and Physiology, University of Göteborg, Göteborg, Sweden
Department of Pediatrics, Pediatric Diabetes Research Center, University of California, San Diego, San Diego, CA, USA
Maike Sander
Department of Molecular Diabetology, University Hospital and Faculty of Medicine, Technische Universität Dresden, Dresden, Germany
Michele Solimena
Paul Langerhans Institute Dresden and German Center for Diabetes Resaerch (DZD e.V.), Helmholtz Center Munich at University Hospital and Faculty of Medicine, Technische Universität Dresden, Dresden, Germany
You can also search for this author in PubMed Google Scholar
Contributions
All authors contributed to the planning and writing of the article and the conception of the figures.
Corresponding authors
Correspondence to Anna L. Gloyn or Michele Solimena .
Ethics declarations
Competing interests.
A.L.G. and A.C.P. are members of the HPAP. A.L.G. is a member of the IIDP. A.L.G. and P.M are members of the Horizon 2020-funded T2DSystems. A.L.G., A.C.P. and M. Sander are members of the HIRN. P.M., M.I. and M. Solimena are members of RHAPSODY and IMIDIA; P.M. and M.I. are members of INNODIA. Through their membership of these consortia, the authors have received financial support as outlined in the acknowledgements.
Peer review
Peer review information.
Nature Metabolism thanks Miriam Cnop, Michael Stitzel and the other, anonymous, reviewer for their contribution to the peer review of this work. Primary Handling Editor: Christoph Schmitt, in collaboration with the Nature Metabolism team.
Additional information
Publisher’s note Springer Nature remains neutral with regard to jurisdictional claims in published maps and institutional affiliations.
Rights and permissions
Springer Nature or its licensor (e.g. a society or other partner) holds exclusive rights to this article under a publishing agreement with the author(s) or other rightsholder(s); author self-archiving of the accepted manuscript version of this article is solely governed by the terms of such publishing agreement and applicable law.
Reprints and permissions
About this article
Cite this article.
Gloyn, A.L., Ibberson, M., Marchetti, P. et al. Every islet matters: improving the impact of human islet research. Nat Metab 4 , 970–977 (2022). https://doi.org/10.1038/s42255-022-00607-8
Download citation
Received : 14 January 2022
Accepted : 14 June 2022
Published : 11 August 2022
Issue Date : August 2022
DOI : https://doi.org/10.1038/s42255-022-00607-8
Share this article
Anyone you share the following link with will be able to read this content:
Sorry, a shareable link is not currently available for this article.
Provided by the Springer Nature SharedIt content-sharing initiative
This article is cited by
Machine-learning-guided recognition of α and β cells from label-free infrared micrographs of living human islets of langerhans.
- Fabio Azzarello
- Francesco Carli
- Francesco Cardarelli
Scientific Reports (2024)
Illuminating the complete ß-cell mass of the human pancreas- signifying a new view on the islets of Langerhans
- Joakim Lehrstrand
- Wayne I. L. Davies
- Ulf Ahlgren
Nature Communications (2024)
Beta cell dysfunction induced by bone morphogenetic protein (BMP)-2 is associated with histone modifications and decreased NeuroD1 chromatin binding
- Adriana Ibarra Urizar
- Michala Prause
- Nils Billestrup
Cell Death & Disease (2023)
Genetic risk converges on regulatory networks mediating early type 2 diabetes
- John T. Walker
- Diane C. Saunders
- Marcela Brissova
Nature (2023)

Quick links
- Explore articles by subject
- Guide to authors
- Editorial policies
Sign up for the Nature Briefing newsletter — what matters in science, free to your inbox daily.

An official website of the United States government
Here’s how you know
Official websites use .gov A .gov website belongs to an official government organization in the United States.
Secure .gov websites use HTTPS A lock ( Lock Locked padlock icon ) or https:// means you’ve safely connected to the .gov website. Share sensitive information only on official, secure websites.
- Entire Site
- Research & Funding
- Health Information
- About NIDDK
- Diabetes Overview
- Insulin, Medicines, & Other Diabetes Treatments
Pancreatic Islet Transplantation
- Español
On this page:
What are islets?
What is pancreatic islet transplantation and how can it treat type 1 diabetes, who are candidates for islet transplantation, how do doctors perform islet transplantation, what are the benefits of islet transplantation, how successful is islet transplantation, what are the risks of islet transplantation, how common is islet transplantation, why isn’t islet transplantation used more often to treat type 1 diabetes, is islet transplantation used to treat any other conditions.
Pancreatic islets, also called islets of Langerhans, are groups of cells in your pancreas . The pancreas is an organ that makes hormones to help your body break down and use food. Islets contain several types of cells, including beta cells that make the hormone insulin. Insulin helps your body use glucose for energy and helps control your blood glucose levels, also called blood sugar.
In people with type 1 diabetes , the body’s immune system attacks and destroys the beta cells. People with type 1 diabetes must take insulin because their bodies no longer make this hormone.
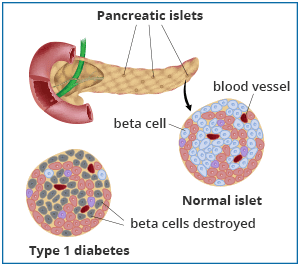
In the type of islet transplantation used to treat type 1 diabetes, also called islet allo-transplantation, doctors take islets with healthy beta cells from the pancreas of a deceased organ donor. Doctors then inject the healthy islet cells taken from the donor into a vein that carries blood to the liver of a person with type 1 diabetes. A person receiving a transplant is called a recipient. These islets begin to make and release insulin in the recipient’s body. More than one injection of transplanted islet cells is often needed to stop using insulin.
For many years, islet transplantation was considered experimental and was only performed in clinical trials. However, in 2023, the U.S. Food and Drug Administration (FDA) approved the first cell therapy made from islets of deceased donors for use in islet transplantation. This cell therapy is approved for adults with type 1 diabetes who have a hard time managing their blood glucose levels and continue to have repeated severely low blood glucose, also called severe hypoglycemia , despite intensive diabetes management and education.
Islet transplantation may help people with type 1 diabetes
- improve their blood glucose levels
- lower or remove the need for insulin injections
- better recognize symptoms of low blood glucose, also called hypoglycemia
- prevent severe hypoglycemia, which is when a person’s blood glucose level becomes so low that he or she needs help from another person to treat the hypoglycemia
A whole pancreas transplant is another procedure that can give a person with type 1 diabetes healthy beta cells. However, a pancreas transplant is a major surgery that carries a greater risk of complications than an islet transplant.
Not all people with type 1 diabetes are good candidates for islet transplantation. Certain people with type 1 diabetes who have blood glucose levels that are difficult to manage, experience severe hypoglycemia, and have hypoglycemia unawareness—a dangerous condition in which a person can’t feel or recognize the symptoms of hypoglycemia—may be candidates.
Doctors consider people for islet transplantation if the possible benefits , such as being better able to reach blood glucose targets without problems such as hypoglycemia, outweigh the risks , such as the possible side effects of immunosuppressants. Immunosuppressants are medicines that recipients must take to prevent their immune system from attacking and destroying the transplanted islets.
People who have type 1 diabetes and have had, or are planning to have, a kidney transplant to treat kidney failure may be candidates for islet transplantation. Islet transplantation may be performed at the same time as or after a kidney transplant. Kidney transplant recipients will already be taking immunosuppressants to prevent rejection of the transplanted kidney. Therefore, the islet transplant does not add much more risk.
Special enzymes are used to remove islets from the pancreas of a deceased donor. The islets are purified and counted in a lab. On average, about 400,000 islets are transplanted in each procedure. 1
The transplant recipient will most often receive a local anesthetic and a sedative—medicine to help you relax—for the procedure. In some cases, the recipient may receive general anesthesia.
The islet transplant infusion procedure involves inserting a thin, flexible tube called a catheter through a small cut in the recipient’s upper abdomen . A radiologist uses x-rays and ultrasound to guide the catheter into the portal vein of the liver . The islets are slowly infused through the catheter and into the liver by gravity. Alternatively, a minimally invasive open procedure can be used to directly visualize a vein near the liver to insert the catheter.
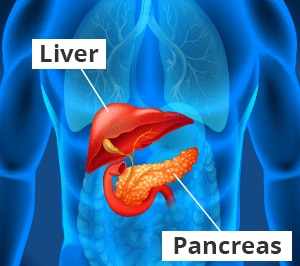
Over the next 2 weeks, new blood vessels form and connect the islets with the blood vessels of the recipient. 2 The beta cells in the islets begin to make and release insulin into the bloodstream immediately after transplant.
Recipients may see the following benefits
- improved blood glucose levels
- less need or no need for insulin injections to manage diabetes
- fewer or no episodes of severe hypoglycemia
- improved awareness of hypoglycemia, which helps prevent episodes of severe hypoglycemia
Research also suggests that islet transplantation may prevent or slow the development of diabetes complications such as heart disease, kidney disease, and nerve or eye damage. 3
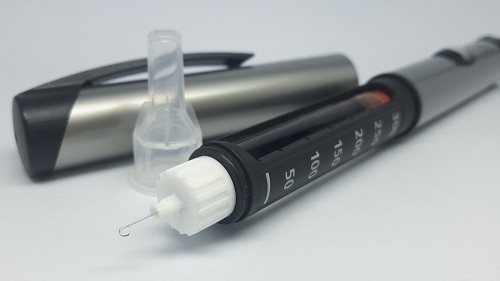
A Phase 3 clinical trial conducted by the National Institutes of Health (NIH)-sponsored Clinical Islet Transplantation Consortium looked at outcomes associated with islet transplantation. Phase 3 trials test new treatments in large groups of people to confirm that treatments are effective and monitor side effects. Participants in this NIH-sponsored study included people with type 1 diabetes with problems managing their blood glucose levels, such as severe hypoglycemia and hypoglycemia unawareness.
The study found that, 1 year after islet transplantation, nearly 9 out of 10 transplant recipients had an A1C level below 7 percent and did not have episodes of severe hypoglycemia. The A1C goal for many people with diabetes is below 7 percent. About half of the recipients did not need to take any insulin. Two years after islet transplantation, about 7 out of 10 recipients had an A1C level of less than 7 percent and did not have episodes of severe hypoglycemia, and about 4 out of 10 did not need insulin. 4
Ongoing research from this study also found that islet transplant recipients experienced significant improvements in their diabetes-related quality of life and reported better overall health status after the transplant. Even transplant recipients who still needed to take insulin to manage their diabetes experienced these improvements. 5
Risks of islet transplantation include
- bleeding, blood clots, and pain after the procedure
- the chance that the transplanted islets may not work well or may stop working
- side effects of anti-rejection medicines, also called immunosuppressants, which are described below
- development of antibodies against the donor cells that may make it more difficult to find an appropriate organ donor if another transplant is needed in the future
Immunosuppressants
After an islet transplant, recipients will take medicines, called immunosuppressants , for as long as the transplanted islets are working. These medicines help prevent the body from rejecting the transplanted islets. Rejection occurs when the body’s immune system sees the islets as “foreign” and tries to destroy them. If the recipient stops taking immunosuppressants, the recipient’s body will reject the transplanted islets, and the islets will stop working.
Immunosuppressants can have many serious side effects. Possible side effects include
- a higher chance of getting infections
- a higher chance of getting cancer
- digestive side effects, such as vomiting , nausea , or diarrhea
- headaches, tremors , or confusion
- high blood pressure
- high blood glucose levels
- high levels of cholesterol and triglycerides in the blood
- kidney damage
Islet transplantation is not a common treatment for type 1 diabetes. In the United States, islet transplantation was considered an experimental treatment for many years and was only performed in clinical trials. With the FDA approval of an islet cell therapy in 2023, islet transplantation is available outside of clinical trials to treat certain adults with type 1 diabetes.
The Collaborative Islet Transplantation Registry (CITR) has reported that, between 1999 and 2015, 1,086 people worldwide received islet transplants for type 1 diabetes. 1 The CITR collects information on islet transplants performed at research centers in North America, Europe, Australia, and Asia. The National Institute of Diabetes and Digestive and Kidney Diseases established and supports the CITR. Breakthrough T1D, formerly known as JDRF or the Juvenile Diabetes Research Foundation, also helped establish CITR.
Islet transplantation is not often used to treat type 1 diabetes because recipients must take immunosuppressants, and donor islets are in short supply.
Islet transplant recipients must take immunosuppressants
Islet transplant recipients must take long-term immunosuppressants, and these medicines may cause serious side effects . Researchers are looking for ways to prevent islet rejection without long-term immunosuppressants. In one approach, called encapsulation, islets are coated with a material that protects them from being attacked by the recipient’s immune system.
Donor islets are in short supply
Only a small number of donor pancreases are available for islet transplantation each year. According to the Organ Procurement and Transplantation Network, 1,315 pancreases were recovered from deceased donors in 2017. Many donated pancreases are not suitable for islet isolation. Also, some donor islets may be damaged or destroyed during the transplant process.
Researchers are studying different ways to overcome the short supply of donor islets. For example, scientists are studying ways to transplant islets from pigs or create new human islets from stem cells .
Doctors may perform a different type of islet transplantation, called islet auto-transplantation, in people who have their whole pancreas removed to treat severe and chronic pancreatitis . People with type 1 diabetes cannot receive islet auto-transplantation.
In islet auto-transplantation, doctors remove a patient’s pancreas, remove the islets from the pancreas, and transplant the islets into the patient’s liver. The goal is to give the body enough healthy islets that make insulin. Patients don’t need to take immunosuppressants after islet auto-transplantation because they receive islets from their own body.
Islet auto-transplantation is not considered experimental.
This content is provided as a service of the National Institute of Diabetes and Digestive and Kidney Diseases (NIDDK), part of the National Institutes of Health. NIDDK translates and disseminates research findings to increase knowledge and understanding about health and disease among patients, health professionals, and the public. Content produced by NIDDK is carefully reviewed by NIDDK scientists and other experts.
The NIDDK would like to thank: Rodolfo Alejandro, M.D., David Baidal, M.D., Ana Alvarez, APRN, Clinical Cell Transplant Program – Diabetes Research Institute, University of Miami Miller School of Medicine

An official website of the United States government
The .gov means it’s official. Federal government websites often end in .gov or .mil. Before sharing sensitive information, make sure you’re on a federal government site.
The site is secure. The https:// ensures that you are connecting to the official website and that any information you provide is encrypted and transmitted securely.
- Publications
- Account settings
- My Bibliography
- Collections
- Citation manager
Save citation to file
Email citation, add to collections.
- Create a new collection
- Add to an existing collection
Add to My Bibliography
Your saved search, create a file for external citation management software, your rss feed.
- Search in PubMed
- Search in NLM Catalog
- Add to Search
From type 1 diabetes biology to therapy: The Human Islet Research Network
Affiliations.
- 1 Department of Diabetes and Cancer Discovery Science, Arthur Riggs Diabetes and Metabolism Research Institute, Beckman Research Institute, City of Hope, Duarte, CA, USA. Electronic address: [email protected].
- 2 Department of Diabetes and Cancer Discovery Science, Arthur Riggs Diabetes and Metabolism Research Institute, Beckman Research Institute, City of Hope, Duarte, CA, USA.
- 3 Diabetes Center, Department of Medicine, University of California, San Francisco, San Francisco, CA, USA.
- 4 Division of Diabetes, Endocrinology and Metabolism, Department of Medicine, Vanderbilt University Medical Center, Nashville, TN, USA.
- 5 Comprehensive Diabetes Center, Department of Medicine, Division of Endocrinology, Diabetes and Metabolism, University of Alabama at Birmingham, Birmingham, AL, USA.
- 6 J. Crayton Pruitt Family, Department of Biomedical Engineering, Herbert Wertheim College of Engineering, University of Florida, Gainesville, FL, USA; University of Florida Diabetes Institute, University of Florida, Gainesville, FL, USA.
- 7 Institute for Diabetes, Obesity, and Metabolism and Department of Medicine, Perelman School of Medicine at the University of Pennsylvania, Philadelphia, PA, USA.
- 8 Chemical Biology and Therapeutics Science Program, Broad Institute, Cambridge, MA, USA.
- PMID: 34224917
- PMCID: PMC8711046
- DOI: 10.1016/j.molmet.2021.101283
Keywords: Beta-cell biology; Human Islet; Immunology; Type 1 Diabetes.
PubMed Disclaimer
Similar articles
- Transdisciplinary approach to restore pancreatic islet function. Fotino C, Molano RD, Ricordi C, Pileggi A. Fotino C, et al. Immunol Res. 2013 Dec;57(1-3):210-21. doi: 10.1007/s12026-013-8437-4. Immunol Res. 2013. PMID: 24233663 Review.
- New prospects for immunotherapy at diagnosis of type 1 diabetes. Pozzilli P, Leslie RD. Pozzilli P, et al. Diabetes Metab Res Rev. 2009 May;25(4):299-301. doi: 10.1002/dmrr.970. Diabetes Metab Res Rev. 2009. PMID: 19405079
- Antigen targets of type 1 diabetes autoimmunity. Roep BO, Peakman M. Roep BO, et al. Cold Spring Harb Perspect Med. 2012 Apr;2(4):a007781. doi: 10.1101/cshperspect.a007781. Cold Spring Harb Perspect Med. 2012. PMID: 22474615 Free PMC article. Review.
- A Path to Insulin Independence: "The End of the Beginning". Stock PG, German MS. Stock PG, et al. Cell Stem Cell. 2016 Apr 7;18(4):431-3. doi: 10.1016/j.stem.2016.03.010. Cell Stem Cell. 2016. PMID: 27058933
- Disease-modifying immunotherapy for the management of autoimmune diabetes. Castro CN, Barcala Tabarrozi AE, Noguerol MA, Liberman AC, Dewey RA, Arzt E, Morelli AE, Perone MJ. Castro CN, et al. Neuroimmunomodulation. 2010;17(3):173-6. doi: 10.1159/000258716. Epub 2010 Feb 4. Neuroimmunomodulation. 2010. PMID: 20134195 Review.
- Untangling the genetics of beta cell dysfunction and death in type 1 diabetes. Robertson CC, Elgamal RM, Henry-Kanarek BA, Arvan P, Chen S, Dhawan S, Eizirik DL, Kaddis JS, Vahedi G, Parker SCJ, Gaulton KJ, Soleimanpour SA. Robertson CC, et al. Mol Metab. 2024 Aug;86:101973. doi: 10.1016/j.molmet.2024.101973. Epub 2024 Jun 22. Mol Metab. 2024. PMID: 38914291 Free PMC article. Review.
- Modeling human T1D-associated autoimmune processes. Khosravi-Maharlooei M, Madley R, Borsotti C, Ferreira LMR, Sharp RC, Brehm MA, Greiner DL, Parent AV, Anderson MS, Sykes M, Creusot RJ. Khosravi-Maharlooei M, et al. Mol Metab. 2022 Feb;56:101417. doi: 10.1016/j.molmet.2021.101417. Epub 2021 Dec 10. Mol Metab. 2022. PMID: 34902607 Free PMC article. Review.
- Campbell-Thompson M., Wasserfall C., Kaddis J., Albanese-O’Neill A., Staeva T., Nierras C., et al. Network for Pancreatic Organ Donors with Diabetes (nPOD): developing a tissue biobank for type 1 diabetes. Diabetes/Metabolism Research and Reviews. 2012;28(7):608–617. - PMC - PubMed
- Kaddis J.S., Olack B.J., Sowinski J., Cravens J., Contreras J.L., Niland J.C. Human pancreatic islets and diabetes research. JAMA. 2009;301(15):1580–1587. - PMC - PubMed
- Bingley P.J., Wherrett D.K., Shultz A., Rafkin L.E., Atkinson M.A., Greenbaum C.J. Type 1 diabetes TrialNet: a multifaceted approach to bringing disease-modifying therapy to clinical use in type 1 diabetes. Diabetes Care. 2018;41(4):653–661. - PMC - PubMed
- Group T.S. The environmental determinants of diabetes in the young (TEDDY) study. Annals of the New York Academy of Sciences. 2008;1150:1–13. - PMC - PubMed
- Nathan D.M. The diabetes control and complications trial/epidemiology of diabetes interventions and complications study at 30 years: overview. Diabetes Care. 2014;37(1):9–16. - PMC - PubMed
Publication types
- Search in MeSH
Related information
Grants and funding.
- U01 DK123559/DK/NIDDK NIH HHS/United States
- UC4 DK112217/DK/NIDDK NIH HHS/United States
- U24 DK104162/DK/NIDDK NIH HHS/United States
- U01 DK120379/DK/NIDDK NIH HHS/United States
- UG3 DK122638/DK/NIDDK NIH HHS/United States
- UH3 DK122638/DK/NIDDK NIH HHS/United States
- U01 DK123717/DK/NIDDK NIH HHS/United States
- P30 DK079626/DK/NIDDK NIH HHS/United States
- U01 CA233363/CA/NCI NIH HHS/United States
LinkOut - more resources
Full text sources.
- ClinicalKey
- Elsevier Science
- Europe PubMed Central
- PubMed Central
- MedlinePlus Health Information
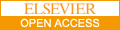
- Citation Manager
NCBI Literature Resources
MeSH PMC Bookshelf Disclaimer
The PubMed wordmark and PubMed logo are registered trademarks of the U.S. Department of Health and Human Services (HHS). Unauthorized use of these marks is strictly prohibited.
- Skip to main content
- Skip to FDA Search
- Skip to in this section menu
- Skip to footer links

The .gov means it’s official. Federal government websites often end in .gov or .mil. Before sharing sensitive information, make sure you're on a federal government site.
The site is secure. The https:// ensures that you are connecting to the official website and that any information you provide is encrypted and transmitted securely.
U.S. Food and Drug Administration
- Search
- Menu
- News & Events
- FDA Newsroom
- Press Announcements
FDA Approves First Cellular Therapy to Treat Patients with Type 1 Diabetes
FDA News Release
Today, the U.S. Food and Drug Administration approved Lantidra, the first allogeneic (donor) pancreatic islet cellular therapy made from deceased donor pancreatic cells for the treatment of type 1 diabetes. Lantidra is approved for the treatment of adults with type 1 diabetes who are unable to approach target glycated hemoglobin (average blood glucose levels) because of current repeated episodes of severe hypoglycemia (low blood sugar) despite intensive diabetes management and education.
“Severe hypoglycemia is a dangerous condition that can lead to injuries resulting from loss of consciousness or seizures,” said Peter Marks, M.D., Ph.D., director of the FDA’s Center for Biologics Evaluation and Research. “Today’s approval, the first-ever cell therapy to treat patients with type 1 diabetes, provides individuals living with type 1 diabetes and recurrent severe hypoglycemia an additional treatment option to help achieve target blood glucose levels.”
Type 1 diabetes is a chronic autoimmune disease that requires lifelong care including requiring insulin, either through multiple daily injections or continuous infusion using a pump, every day to live. People with type 1 diabetes also perform blood glucose checks several times a day to guide the management of their diabetes.
Some people with type 1 diabetes have trouble managing the amount of insulin needed every day to prevent hyperglycemia (high blood sugar) without causing hypoglycemia. They may also develop hypoglycemia unawareness, where they are unable to detect their blood glucose is dropping and may not have a chance to treat themselves to prevent their blood glucose from further dropping. This makes it difficult to dose insulin. Lantidra provides a potential treatment option for these patients.
The primary mechanism of action of Lantidra is believed to be the secretion of insulin by the infused allogeneic islet beta cells. In some patients with type 1 diabetes, these infused cells can produce enough insulin, so the patient no longer needs to take insulin (by injections or pump) to control their blood sugar levels. Lantidra is administered as a single infusion into the hepatic (liver) portal vein. An additional infusion of Lantidra may be performed depending on the patient’s response to the initial dose.
The safety and effectiveness of Lantidra was evaluated in two non-randomized, single-arm studies in which a total of 30 participants with type 1 diabetes and hypoglycemic unawareness received at least one infusion and a maximum of three infusions. Overall, 21 participants did not need to take insulin for a year or more, with 11 participants not needing insulin for one to five years and 10 participants not needing insulin for more than five years. Five participants did not achieve any days of insulin independence.
Adverse reactions associated with Lantidra varied with each participant depending on the number of infusions they received and the length of time they were followed and may not reflect the rates observed in practice The most common adverse reactions included nausea, fatigue, anemia, diarrhea and abdominal pain. A majority of participants experienced at least one serious adverse reaction related to the procedure for infusing Lantidra into the hepatic portal vein and the use of immunosuppressive medications needed to maintain the islet cell viability. Some serious adverse reactions required discontinuation of immunosuppressive medications, which resulted in the loss of islet cell function and insulin independence. These adverse events should be considered when assessing the benefits and risks of Lantidra for each patient. Lantidra is approved with patient-directed labeling to inform patients with type 1 diabetes about benefits and risks of Lantidra.
The FDA granted approval of Lantidra to CellTrans Inc.
The FDA, an agency within the U.S. Department of Health and Human Services, protects the public health by assuring the safety, effectiveness, and security of human and veterinary drugs, vaccines and other biological products for human use, and medical devices. The agency also is responsible for the safety and security of our nation’s food supply, cosmetics, dietary supplements, products that give off electronic radiation, and for regulating tobacco products.
- U.S. Department of Health & Human Services

- Virtual Tour
- Staff Directory
- En Español
You are here
News releases.
News Release
Wednesday, March 21, 2018
Islet transplantation improves quality of life for people with hard-to-control type 1 diabetes
NIH-funded study finds consistent, dramatic improvements among clinical trial participants.
Quality of life for people with type 1 diabetes who had frequent severe hypoglycemia — a potentially fatal low blood glucose (blood sugar) level — improved consistently and dramatically following transplantation of insulin-producing pancreatic islets , according to findings published online March 21 in Diabetes Care. The results come from a Phase 3 clinical trial funded by the National Institute of Allergy and Infectious Diseases (NIAID) and the National Institute of Diabetes and Digestive and Kidney Diseases (NIDDK), both part of the National Institutes of Health.
The greatest improvements were seen in diabetes-related quality of life. Islet recipients also reported better overall health status after transplant, despite the need for lifelong treatment with immune-suppressing drugs to prevent transplant rejection. Researchers observed these improvements even among transplant recipients who still required insulin therapy to manage their diabetes.
The trial enrolled 48 people with type 1 diabetes who had hypoglycemia unawareness — an impaired ability to sense drops in blood glucose levels — and experienced frequent episodes of severe hypoglycemia despite receiving expert care.
Previously reported clinical outcomes from the trial showed that islet transplantation prevents severe hypoglycemia and improves blood glucose awareness and control. The study was conducted by the NIH-funded Clinical Islet Transplantation Consortium .
“Although insulin therapy is life-saving, type 1 diabetes remains an extremely challenging condition to manage,” said NIAID Director Anthony S. Fauci, M.D. “For people unable to safely control type 1 diabetes despite optimal medical management, islet transplantation offers hope for improving not only physical health but also overall quality of life.”
Pancreatic islets release the hormone insulin, which helps control blood glucose levels. In type 1 diabetes , the body’s immune system attacks and destroys the insulin-producing cells in islets. People with the disease must take insulin to live, but insulin injections or pumps cannot control blood glucose levels as precisely as insulin released naturally from the pancreas. Even with diligent monitoring, blood glucose can often reach levels that are higher or lower than normal.
A low blood glucose level, or hypoglycemia, typically is accompanied by tremors, sweating, nausea and/or heart palpitations. These symptoms prompt the person to eat or drink to raise their blood glucose. However, some people do not experience these early warning signs. This impaired awareness of hypoglycemia raises the risk of potentially life-threatening severe hypoglycemic events, during which the person is unable to treat himself or herself. These episodes can lead to accidents, injuries, coma and death.
“People with type 1 diabetes who are at high risk for hypoglycemic events have to practice caution every moment, even while sleeping. It is an exhausting endeavor that—like the events themselves—can keep them from living full lives,” said NIDDK Director Griffin P. Rodgers, M.D. “Although islet transplantation remains experimental, we are very encouraged by these findings, as we are by the rapid improvements in other treatments to help people with type 1 diabetes monitor and manage their blood glucose, including artificial pancreas technology .”
All 48 study participants received at least one islet transplant. One year after their first transplant, 42 participants (88 percent) were free of severe hypoglycemic events, had established near-normal blood glucose control, and had restored awareness of hypoglycemia. Only a small number of functional insulin-producing cells are necessary to restore hypoglycemic awareness, but this amount may not be sufficient to fully regulate a person’s blood glucose levels. Approximately half of the transplant recipients needed to continue taking insulin to control their blood glucose levels.
The study design incorporated four well-established, commercially available quality-of-life surveys that were given to participants repeatedly before and after islet transplantation. Two of the surveys were specific for diabetes, while two assessed health more generally.
“This study was very rigorous both in terms of the number of measures used to assess quality of life and the number of evaluations performed,” said paper co-author Nancy D. Bridges, M.D., chief of the Transplantation Branch at NIAID. “Islet transplant recipients not only reported a decrease in concerns and fears related to their diabetes, but also felt better overall, despite the need to take daily immunosuppressive drugs to prevent transplant rejection.”
Reported improvements in quality of life were similar among islet recipients who still needed to take insulin to manage their diabetes and those who did not. The researchers concluded that elimination of severe hypoglycemia and the associated fears accounted for these improvements, appearing to outweigh concerns about the need to continue insulin injections.
Islet transplantation is an investigational therapy in the United States. While promising for people whose type 1 diabetes cannot be controlled with standard treatments, the procedure is not appropriate for most people with type 1 diabetes, as there are risks associated with the transplant procedure, such as bleeding, as well as side effects of immunosuppressive medications, such as decreased kidney function and increased susceptibility to infections.
In the NIH-funded trial, investigators at eight study sites in North America used a standardized manufacturing protocol to prepare purified islets from the pancreases of deceased human donors. The study was designed, after discussions with the U.S. Food and Drug Administration, to provide evidence to support licensure of the manufactured islet product. NIAID, the regulatory sponsor of the study, has submitted final reports and clinical trial data to FDA, laying the groundwork for individual universities and companies to submit biologics license applications for the manufacture of purified human pancreatic islets.
For more information about the study, see ClinicalTrials.gov using identifier NCT00434811 . The study was funded by NIAID and NIDDK, both components of NIH, under grant numbers U01AI089317, U01AI089316, U01AI065191, U01DK085531, U01DK070431, U01DK070431, U01DK070460, U01AI065193, U01DK070430 and U01AI065192. The work was partially supported by the National Center for Advancing Translational Sciences, NIH, under grant numbers UL1TR000454, UL1RR025741, UL1TR000150, UL1TR000004, UL1TR000050, UL1TR000460, M01RR000400, UL1TR000114, M01RR00040 and UL1TR000003.
NIAID conducts and supports research — at NIH, throughout the United States, and worldwide — to study the causes of infectious and immune-mediated diseases, and to develop better means of preventing, diagnosing and treating these illnesses. News releases, fact sheets and other NIAID-related materials are available on the NIAID website .
The NIDDK, part of the NIH, conducts and supports basic and clinical research and research training on some of the most common, severe, and disabling conditions affecting Americans. The Institute’s research interests include: diabetes and other endocrine and metabolic diseases; digestive diseases, nutrition, and obesity; and kidney, urologic, and hematologic diseases. For more information, visit www.niddk.nih.gov .
About the National Institutes of Health (NIH): NIH, the nation's medical research agency, includes 27 Institutes and Centers and is a component of the U.S. Department of Health and Human Services. NIH is the primary federal agency conducting and supporting basic, clinical, and translational medical research, and is investigating the causes, treatments, and cures for both common and rare diseases. For more information about NIH and its programs, visit www.nih.gov .
NIH…Turning Discovery Into Health ®
Connect with Us
- More Social Media from NIH
- Fierce Pharma
- Fierce Biotech
- Fierce Healthcare
- Fierce Life Sciences Events
- Cell & Gene Therapy
- Clinical Data
- Venture Capital
- Diagnostics
- AI and Machine Learning
- Special Reports
- Clinical Development
- Special Report
- Awards Gala
- Fierce Events
- Industry Events
- Whitepapers
Islet-homing engineered T regs protect against Type 1 diabetes
Like many autoimmune diseases, Type 1 diabetes stems from effector T cells gone rogue. The immune cells launch an attack on the insulin-producing beta islet cells in the pancreas, leaving patients without the ability to process glucose. As a result, patients require lifelong insulin treatment.
With the advent of T-cell therapies, scientists have been looking to the body’s own defense against renegade T effector cells to see if they can prevent or even reverse Type 1 diabetes. In a study published Oct. 5 in Science Translational Medicine, researchers from Benaroya Research Institute and Seattle Children’s Research Institute described how they engineered regulatory T cells—or Tregs, cells that police the immune system if it begins attacking host tissues—that homed to the pancreas, where they successfully blocked diabetes development in mice.
“This study is an important step because it shows that by copying a natural process that protects the body, we might be able to stop [Type 1 diabetes] at the source,” Soo Jung Yang, Ph.D., first author, said in a press release .
The scientists were building on a decade of their own work genetically engineering Tregs to treat autoimmune disease, with a specific focus on Type 1 diabetes. Previously, they had developed a class of Tregs that expressed the FOXP3 gene, defects in which have been linked to their demise and, thus, inability to control effector T-cell activity. Their engineered Tregs had successfully suppressed effector T cells, but the scientists wanted to make them more specific to the pancreas’ beta islet cells—ideally sidestepping the potential problem of widespread immunosuppression, along with improving their efficacy.
To that end, the researchers combined two approaches to create engineered Tregs that were specific to an antigen on beta islet cells: the same platform they had developed to enhance FOXP3 gene expression in the Tregs, plus human T-cell receptor gene transfer. For the latter, they used seven different islet-specific T-cell receptors, all of which had been derived from effector T cells that were isolated from patients with Type 1 diabetes.
After confirming that the antigen-specific engineered Tregs would suppress effector T cells in the lab, the researchers moved to testing the cells in mice. They mixed the engineered Tregs with effector T cells and injected them into mouse models that would develop Type 1 diabetes upon administration of effector T cells, then observed their blood glucose levels over 49 days. The mice that received the engineered Tregs were almost completely diabetes-free by the end of the period, while those that were injected with a control solution all developed the condition between days 9 and 15.
Importantly, the engineered Tregs also needed to persist in the pancreatic tissue to have a long-term therapeutic benefit. In the mouse models, they had: The scientists found that by the end of the period, the antigen-specific engineered Tregs were as abundant in the treated mice as natural Tregs in healthy subjects.
While the scientists are well on their way to taking the research to the clinic, co-corresponding author and Benaroya Research Institute President Dr. Jane Buckner, MD, told Fierce in an email, there is a bit more work to be done. For one, while the engineered Tregs did indeed work, they had different levels of effector T-cell suppression depending on which of the T-cell receptors had been used to create them.
“What we found most surprising was the difference in the level of suppression seen with different TCRs, even those that were specific for the same peptide,” she said. “This suggests to us that better understanding the characteristics of TCRs that influence the function of engineered Tregs will be important to inform selection of TCRs for therapy.”
In addition, the scientists so far have developed their engineered Tregs specifically for patients who carry the HLA DR0401 allele, one of several HLA alleles associated with Type 1 diabetes. Going forward, they plan to identify and test T-cell receptors restricted to other HLA types, Dr. Buckner said.
In the meantime, companies are making progress on tackling Type 1 diabetes from other angles. Vertex made headlines last year with the first "functional cure” of the condition in a man who was infused with insulin-producing beta islet cells grown from stem cells. On another front, Provention Bio is looking to gain FDA approval next month for its drug teplizumab, a humanized anti-CD3 monoclonal antibody that’s designed to delay the onset of diabetes by a median of two years in high-risk children and adults.
Diabetes Qualified

Consensus guidance for monitoring individuals with islet autoantibody-positive pre-stage 3 type 1 diabetes
A guidance document, co-authored by 66 international experts including Australian researchers, has been endorsed by key diabetes organisations, including the Australian Diabetes Society (ADS). The consensus report outlines the best methods and recommendations for the frequency of monitoring progression of type 1 diabetes across pre-symptomatic stages. It also provides guidance on when insulin treatment should be introduced, for children, adults and in pregnancy. Credentialled diabetes educator Carolien Koreneff explains.
Consensus guidance for monitoring individuals with islet autoantibody-positive pre-stage 3 type 1 diabetes
Type 1 diabetes is usually diagnosed when high blood glucose levels cause the symptoms of hyperglycaemia, including thirst, frequent urination, fatigue and weight loss. This means that the diagnosis often ensues at a point of critical illness, requiring urgent hospital admission due to diabetic ketoacidosis (DKA), particularly in children. However, research has shown that the auto-immune condition starts well before the obvious symptoms are present.
Screening and monitoring of type 1 diabetes will bring forward diagnoses and will make early detection a reality. To this day, there was no standard advice on how to detect, follow up or support people with, what is now referred to as, early-stage or pre-symptomatic type 1 diabetes. But this is all about to change.
Stages of type 1 diabetes
Previously it was thought that type 1 diabetes develops shortly before the hyperglycaemia, but years of research have shown that there are some detectable changes in people before the symptoms develop, for example in beta cell mass and insulin production. These changes can occur months or even years before the diagnosis.
If a person is genetically susceptible, environmental factors (such as viral infections) can trigger their immune system to start attacking the pancreas. The body will produce auto-antibodies when there is such an immune attack against the beta cells. These antibodies can be detected in the blood. There are several antibodies associated with type 1 diabetes including:
- Insulinoma-associated protein 2 autoantibody (IA-2A)
- Insulin autoantibodies (IAA)
- Islet cell autoantibodies (ICA)
- Glutamic acid decarboxylase 65 autoantibody (GAD-65)
- Zinc transporter 8 autoantibody (ZnT8)
A person at risk of, or recently diagnosed with, type 1 diabetes will have auto-antibodies. It is estimated that people with two or more auto-antibodies have a 70% chance of developing type 1 diabetes within the next decade and nearly 100% chance of developing type 1 diabetes in their lifetime. In other words, having auto-antibodies can predict if a person will go on to develop the condition.
The Juvenile Diabetes Research Foundation (JDRF) have described the development of type 1 diabetes in the following stages:
No diabetes: There are plenty of beta cells (a ‘normal’ level) in the pancreas that produce enough insulin to maintain normoglycaemia.
Triggering event causes the immune system to attack beta cells – auto-antibodies are produced and are detectable in blood tests.

- Two or more auto-antibodies can be found, but the person’s glucose levels remain in the ‘normal’ range.

- Two or more auto-antibodies can be found, and blood glucose levels have started to rise above target.
(Both stage 1 and stage 2 are referred to as pre-symptomatic stages.)

- Stage 3: Two or more auto-antibodies can be found, and blood glucose levels are high as there is now significant beta cell loss. This is when the diagnosis of type 1 diabetes is made, and insulin treatment is introduced.

- The person has longstanding type 1 diabetes after numerous years. Two or more auto-antibodies may or may not be detected in the blood. Long-term health complications can occur.
Although people in stages 1 and 2 don’t officially have diabetes, they are at increased risk of developing type 1 diabetes down the track. This is similar to people with pre-diabetes who are at great risk of developing type 2 diabetes over time.
Screening for type 1 diabetes
By detecting autoantibodies in the blood, through a simple blood test, the chance of someone going on to develop type 1 diabetes can be determined. If the chance is high, they can be monitored and it may reduce the shock of the diagnosis, at least to some degree. Perhaps more importantly, through screening, health checks, and education, the rate of developing diabetic ketoacidosis (DKA) can be reduced by 90%.
Through research it may be possible over time to identify which environmental events are associated with the development of type 1 diabetes, and to develop preventative strategies for those who are genetically susceptible. Screening for type 1 diabetes risk can therefore also identify people who may be eligible to enter clinical trials, such as the Australian Type 1 Diabetes National Screening Pilot and the Type 1 Screen , both led by Associate Professor John Wentworth and Dr Kristine Bell, who also co-authored the guidance document.
It is expected that, once widely implemented, screening will identify significant numbers of auto-antibody positive children and adults at risk of or living with early-stage type 1 diabetes.
The consensus report
JDRF, in conjunction with international experts and societies, recommend the following:
- Partnerships should be fostered between endocrinologists and primary-care providers to care for people who are islet autoantibody positive (IAb+).
- When people who are IAb+ are initially identified, there is a need for confirmation using a second sample.
- Single IAb+ individuals are at lower risk of progression than multiple IAb+ individuals.
- Individuals with early-stage type 1 diabetes should have periodic medical monitoring, including regular assessments of glucose levels, regular education about symptoms of diabetes and DKA, and psychosocial support.
- Interested people with stage 2 type 1 diabetes should be offered trial participation or approved therapies.
- All health professionals involved in monitoring and care of individuals with type 1 diabetes have a responsibility to provide education.
The guidance also emphasises “significant unmet needs for further research on early-stage type 1 diabetes to increase the rigour of future recommendations and inform clinical care”.
The authors also state that “it is important to note that there are very limited data in adults aged 45 years and older who are IAb+” and that “this consensus document does not encompass screening for islet autoantibodies, and only provides expert clinical advice for monitoring of individuals who have screened positive for at least one islet autoantibody”.
More information
If you would like to read the full consensus report, please click this link .
References:
- Phillip, M., Achenbach, P., Addala, A. et al. Consensus guidance for monitoring individuals with islet autoantibody-positive pre-stage 3 type 1 diabetes. Diabetologia (2024). https://doi.org/10.1007/s00125-024-06205-5
- The stages of type 1 diabetes by JDRF. https://jdrf.org.au/stages-of-type-1-diabetes/ accessed 24 th July 2024
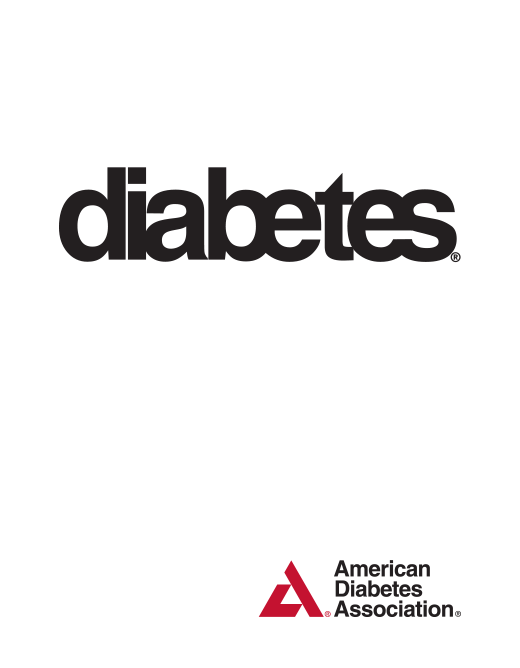
- Previous Article
- Next Article
RESEARCH DESIGN AND METHODS
Acknowledgments, enterovirus infection and progression from islet autoimmunity to type 1 diabetes : the diabetes and autoimmunity study in the young (daisy).
- Split-Screen
- Article contents
- Figures & tables
- Supplementary Data
- Peer Review
- Open the PDF for in another window
- Cite Icon Cite
- Get Permissions
Lars C. Stene , Sami Oikarinen , Heikki Hyöty , Katherine J. Barriga , Jill M. Norris , Georgeanna Klingensmith , John C. Hutton , Henry A. Erlich , George S. Eisenbarth , Marian Rewers; Enterovirus Infection and Progression From Islet Autoimmunity to Type 1 Diabetes : The Diabetes and Autoimmunity Study in the Young (DAISY) . Diabetes 1 December 2010; 59 (12): 3174–3180. https://doi.org/10.2337/db10-0866
Download citation file:
- Ris (Zotero)
- Reference Manager
To investigate whether enterovirus infections predict progression to type 1 diabetes in genetically predisposed children repeatedly positive for islet autoantibodies.
Since 1993, the Diabetes and Autoimmunity Study in the Young (DAISY) has followed 2,365 genetically predisposed children for islet autoimmunity and type 1 diabetes. Venous blood and rectal swabs were collected every 3–6 months after seroconversion for islet autoantibodies (against GAD, insulin, or insulinoma-associated antigen-2 [IA-2]) until diagnosis of diabetes. Enteroviral RNA in serum or rectal swabs was detected using reverse transcriptase PCR with primers specific for the conserved 5′ noncoding region, detecting essentially all enterovirus serotypes.
Of 140 children who seroconverted to repeated positivity for islet autoantibodies at a median age of 4.0 years, 50 progressed to type 1 diabetes during a median follow-up of 4.2 years. The risk of progression to clinical type 1 diabetes in the sample interval following detection of enteroviral RNA in serum (three diabetes cases diagnosed among 17 intervals) was significantly increased compared with that in intervals following a negative serum enteroviral RNA test (33 cases diagnosed among 1,064 intervals; hazard ratio 7.02 [95% CI 1.95–25.3] after adjusting for number of autoantibodies). Results remained significant after adjustment for ZnT8-autoantibodies and after restriction to various subgroups. Enteroviral RNA in rectal swabs was not predictive of progression to type 1 diabetes. No evidence for viral persistence was found.
This novel observation suggests that progression from islet autoimmunity to type 1 diabetes may increase after an enterovirus infection characterized by the presence of viral RNA in blood.
Type 1 diabetes results from destruction of the insulin-producing β-cells in the pancreatic islets ( 1 ). The majority of patients carry the HLA DRB1 * 03-DQB1 * 0201 or DRB1 * 04-DQB1 * 0302 susceptibility haplotype or both, but these are not sufficient for development of disease. For many years, viral infections have been suspected to play a role, but the specific etiologic agent(s) in human type 1 diabetes remains elusive. While several viruses have been linked to type 1 diabetes, seroepidemiology, histopathology, animal studies, and in vitro experiments have provided the strongest overall evidence for enteroviruses, although results have been somewhat conflicting and not conclusive ( 2 , – 4 ).
Autoantibodies to islet autoantigens are present for years prior to diagnosis of type 1 diabetes ( 1 ), and prospective studies testing whether enterovirus could predict islet autoantibodies have yielded conflicting results, with positive results in Finnish studies ( 5 , – 7 ) and no association found elsewhere ( 8 , 9 ).
Results from animal models suggested that viral infections usually cannot initiate the autoimmune disease process leading to diabetes but may accelerate an already initiated disease process. Studies in various strains of NOD mice have shown that enteroviral infections may accelerate the progression to diabetes only if they occur after autoreactive T-cells have already accumulated in the islets ( 10 , , – 13 ). In an attempt to evaluate for the first time whether such a general model of disease progression rather than initiation by enteroviruses applies to human type 1 diabetes, we tested whether enteroviral infections predict progression to type 1 diabetes in children repeatedly positive for islet autoantibodies.
From 1993 to 2004, children born at St. Joseph's Hospital in Denver carrying HLA genotypes that confer increased risk for type 1 diabetes and siblings or offspring of people with type 1 diabetes (regardless of their genotype), identified from the Barbara Davis Center for Childhood Diabetes, were enrolled in the Diabetes Autoimmunity Study in the Young (DAISY). Informed consent was obtained from parents of all children, and the study was approved by the Colorado Multiple Institutional Review Board. Children were followed longitudinally from soon after birth and screened for islet autoantibodies at ages 9, 15, and 24 months and annually thereafter. Siblings or offspring of individuals with type 1 diabetes were enrolled after 9 months of age (median age 1.33 years [range 0.02–7.9]). Children who tested positive for islet autoantibodies were scheduled for more frequent follow-up, with visits at 3–6 month intervals.
The current study is a cohort analysis of all children who tested positive for one or more islet autoantibody on two or more consecutive clinic visits and provided at least one sample for enterovirus testing during follow-up for type 1 diabetes. Figure 1 shows a flow chart illustrating how the study cohort was formed. A batch of samples collected 1993–2004 was sent for enterovirus testing in 2005, and another batch of samples collected during 2005–June 2007 was sent in 2008. The children were further followed for diagnosis of type 1 diabetes, and the current analysis includes information on autoantibody status and diabetes up to April 2009. Type 1 diabetes was clinically diagnosed based on American Diabetes Association criteria ( 14 ), and details of procedures and clinical characteristics have been described elsewhere ( 15 , 16 ).
Laboratory methods.
HLA genotyping was done at Roche Molecular Systems, Alameda, CA, as previously described ( 17 ). Children with genotypes DRB1 * 04-DQB1 * 0302/DRB1 * 03-DQB1 * 0201 were defined as high risk, and DRB1 * 04-DQB1 * 0302/DRB1 * 04-DQB1 * 0302 or DRB1 * 03/ * 03 or DRB1 * 04-DQB1 * 0302/X (where X is not DRB1 * 04 , DQB1 * 0302, DRB1 * 03 , or DR2,DQB1 * 0602 ) was categorized as conferring moderate risk for type 1 diabetes.
At each clinic visit, venous blood and rectal swabs were collected. Blood samples were immediately processed, aliquoted, and stored at −70°C until testing. Rectal swabs were immediately placed in 1 ml transport medium (veal infusion broth or M4−3 medium) and stored at −70°C as previously described ( 8 ). Radioimmunoassays were used to measure serum autoantibodies to insulin, GAD 65 , and IA-2 (BDC512) in George Eisenbarth's laboratory as previously described ( 18 , , – 21 ), with rigorous duplicate testing and confirmation of all positive and a subset of negative results ( 22 ). ZnT8 autoantibodies were measured in John Hutton's laboratory, as previously described, using a dimeric construct incorporating monomeric forms of the COOH-terminus with the polymorphic 325 Arg and Trp variants joined by a flexible linker ( 23 , 24 ). This autoantibody was measured in stored, available samples (81% of samples with valid serum enterovirus RNA measurements).
All enterovirus assays were carried out in Heikki Hyöty's laboratory at the University of Tampere. All virus analyses were done blindly, without knowledge of the disease status of the child. RNA was extracted from 140 μl serum and from 140 μl rectal swab solution according to the manufacturer's protocol (QIAamp viral RNA kit; Qiagen, Hilden, Germany). The presence of enterovirus RNA was detected with RT-PCR using primers specific for the 5′ noncoding region conserved among Picornaviridae and subsequent enterovirus-specific hybridization with lanthanide chelated probes, providing sensitive and specific detection of practically all known enterovirus serotypes ( 25 ). All samples with a RT-PCR signal five-fold or higher than a negative control were tested two more times, and a sample was interpreted as positive if at least two out of the three tests were five-fold or higher than the negative control. The 5′ noncoding region of detected enteroviruses was partially sequenced, and sequences were analyzed as described in detail in the online appendix ( http://diabetes.diabetesjournals.org/cgi/content/full/db10-0866/DC1 .
Enterovirus antibodies were measured in the batch of sera collected 1993–2004, using enzyme immunoassay as described previously ( 26 , – 28 ). Antibodies tested were immunoglobulin M antibodies against an antigen cocktail containing coxsackievirus B3, A16, and echovirus 11, as well as IgA and IgG antibodies against purified coxsackievirus B4 and a synthetic enterovirus peptide antigen, KEVPALTVETGAT-C, derived from an immunodominant region of capsid protein VP1 ( 29 ), which is a common epitope for many enteroviruses ( 30 ). The purified viruses were heat treated to expose antigenic determinants common for various enterovirus serotypes ( 26 ).
Definition of infection.
Our primary, a priori definition of infection at a given visit was positivity (as defined above) for RT-PCR detection of enterovirus RNA in serum or rectal swab. Additional analyses were done separately for serum PCR and rectal PCR and for the subset of samples tested for enterovirus antibodies. A sample was defined as positive for serology if there was a twofold or higher increase in level (optical density value) of any of the measured antibodies in the subsequent visit (samples usually 3–6 months apart), with an additional requirement that the signal-to-background ratio should exceed three. Additional two-fold or higher increases in enterovirus antibodies in a third (or later) consecutive sample drawn within 9 months of a previous one were not counted as an additional infection. These criteria were the same as those used in our previous prospective studies ( 6 ).
Statistical analysis.
Using Cox regression, we compared the rate of progression to type 1 diabetes under two different models, which we have called the rapid effect model and the cumulative effect model. Both treat enterovirus infection as a time-dependent variable. In the rapid effect model, we estimated the rate of progression to diabetes in the sample interval following detection of enterovirus (median 4 months) compared with sample intervals where enterovirus was not detected. The exposure status returned to zero at the next clinic visit unless enterovirus was also found here. In the cumulative effect model, we estimated the rate of progression to diabetes according to the cumulative number of infections acquired during follow-up, which also allows for detection of delayed effects. Each individual first contributed follow-up time with zero infections, and the exposure variable increased by one at each visit when a new infection was detected. Because few individuals had repeated infections, the cumulative exposure variable had to be grouped (0 vs. ≥1 for serum RNA; 0, 1, and ≥2 for rectal swab RNA). The main time variable was time from the first clinic visit at which a child tested positive for islet autoantibodies to type 1 diabetes diagnosis or to the most recent visit (up to 9 April 2009) at which the child was known not to have diabetes. Because enteroviral RNA was relatively rarely detected in serum and, consequently, the number of events during the exposed periods were limited, we also carried out a Monte Carlo permutation test with 10,000 repeated permutations of the enterovirus variable to assess the validity of the standard inference based on the Cox regression model. All analyses were done using Stata, version 11 (StataCorp, College Station, TX). A 95% CI for the hazard ratio excluding the value 1.00 or a P value <0.05 was regarded as statistically significant.
A total of 140 children seroconverted for islet autoantibodies at a median age of 4.0 years. Of those, 50 developed type 1 diabetes at a median age of 8.7 years after a median follow-up of 4.1 years from the initial appearance of islet autoantibodies ( Table 1 ). The samples tested for enterovirus were collected prior to June 2007, and 41 of the 50 children had developed type 1 diabetes by that time while another nine progressed to type 1 diabetes between June 2007 and April 2009 ( Fig. 1 ).
Characteristics of the cohort and results of Cox regression survival analysis of progression from islet autoimmunity to type 1 diabetes
. | Progressed to type 1 diabetes ( = 50) . | No type 1 diabetes ( = 90) . | Unadjusted HR (95% CI) . | Adjusted HR (95% CI) . |
---|---|---|---|---|
Age (years) at diagnosis of type 1 diabetes | 8.7 (1.9–15) | |||
Follow-up (years) from onset of islet autoimmunity | 4.1 (0.2–11) | 4.6 (1.6–14) | ||
Positive for >2 islet autoantibodies at the first and/or second positive visit | 36 (72%) | 21 (23.3%) | 4.57 (2.46–8.51) | 4.24 (2.26–7.95) |
Female sex | 26 (52%) | 48 (53.3%) | 1.18 (0.67–2.06) | 1.41 (0.79–2.50) |
First-degree relative with type 1 diabetes | 35 (70%) | 53 (58.9%) | 1.23 (0.67–2.26) | 1.13 (0.61–2.10) |
* * * * | 26 (52.0%) | 27 (30.0%) | 1.84 (1.06–3.21) | 1.51 (0.86–2.67) |
Non–Hispanic white ethnicity | 46 (92.0%) | 72 (80.0%) | 1.94 (0.70–5.39) | 1.45 (0.51–4.13) |
Age (years) when first islet autoantibody positive | 3.1 (0.7–12) | 5.2 (0.7–13) | 0.93 (0.85–1.02) | 1.01 (0.091–1.11) |
. | Progressed to type 1 diabetes ( = 50) . | No type 1 diabetes ( = 90) . | Unadjusted HR (95% CI) . | Adjusted HR (95% CI) . |
---|---|---|---|---|
Age (years) at diagnosis of type 1 diabetes | 8.7 (1.9–15) | |||
Follow-up (years) from onset of islet autoimmunity | 4.1 (0.2–11) | 4.6 (1.6–14) | ||
Positive for >2 islet autoantibodies at the first and/or second positive visit | 36 (72%) | 21 (23.3%) | 4.57 (2.46–8.51) | 4.24 (2.26–7.95) |
Female sex | 26 (52%) | 48 (53.3%) | 1.18 (0.67–2.06) | 1.41 (0.79–2.50) |
First-degree relative with type 1 diabetes | 35 (70%) | 53 (58.9%) | 1.23 (0.67–2.26) | 1.13 (0.61–2.10) |
* * * * | 26 (52.0%) | 27 (30.0%) | 1.84 (1.06–3.21) | 1.51 (0.86–2.67) |
Non–Hispanic white ethnicity | 46 (92.0%) | 72 (80.0%) | 1.94 (0.70–5.39) | 1.45 (0.51–4.13) |
Age (years) when first islet autoantibody positive | 3.1 (0.7–12) | 5.2 (0.7–13) | 0.93 (0.85–1.02) | 1.01 (0.091–1.11) |
Data are median (range) or n (%) unless otherwise indicated.
†Estimates from Cox regression model simultaneously adjusting for multiple autoantibodies in first two visits, HLA high risk genotype, presence of first-degree relative with type 1 diabetes, and age when first positive for islet autoantibodies.
‡Of these, 35 had an affected father only, 16 had an affected mother only, 34 had an affected sibling, and 3 had a sibling and a parent with type 1 diabetes.
§Ethnic group was self-reported. There were 118 non–Hispanic whites, 19 Hispanics, one African American, and two children of mixed ethnicity in the cohort.
¶HRs per year increase in age when first positive for islet autoantibodies.
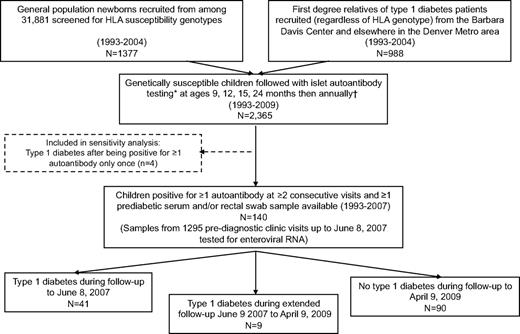
Flow chart illustrating formation of the study cohort. *Samples were tested for the three islet autoantibodies: anti-GAD65, anti-insulin, and anti–IA-2. †If positive for ≥1 islet autoantibody at or after 12 months of age, frequency of blood sampling was increased to every 3–6 months.
Positivity for two or more islet autoantibodies at the first or second positive visit strongly predicted progression to type 1 diabetes, independent of other factors ( Table 1 ). Those who progressed to type 1 diabetes tended to more often carry the high-risk HLA genotype, to have a first-degree relative with type 1 diabetes, and to seroconvert for islet autoantibodies at an earlier age, but these factors did not significantly predict progression to type 1 diabetes independently of positivity for multiple islet autoantibodies in at least one of the two first positive visits ( Table 1 ). The number of positive islet autoantibodies treated as a time-dependent variable was also highly predictive of progression to type 1 diabetes, and positivity for ZnT8-autoantibodies significantly predicted progression both before and after adjusting for the other three islet autoantibodies (supplemental Table 1).
Enterovirus infections.
Enteroviral RNA results were available from serum and rectal swabs collected at 1,081 and 1,242 prediagnostic clinic visits, respectively. Results were available for either serum or rectal swab at 1,295 visits and from both types of specimens at 1,028 visits. The median interval between the visits was 4 months. Enteroviral RNA was detected at a total of 54 of 1,295 visits (4.2%). At eight of these 54 visits, enteroviral RNA was detected in both serum and rectal swab. Of the 140 children in the cohort, 31 (22.1%) had at least one serum or rectal swab sample positive for enteroviral RNA. While 19 of these 31 were positive only once, some had up to six positive visits. Only two children were ever positive twice for serum enteroviral RNA.
The prevalence of enterovirus RNA in serum or rectal swabs declined with age from nearly 10% for the age-group <2.5 years to ∼1% for the age-group ≥7.5 years (supplemental Fig. 1). Enteroviral RNA tended to be more frequent in boys and at visits positive for multiple islet autoantibodies, but these differences were mostly of borderline statistical significance and not consistent among serum and rectal swab samples (supplemental Table 2). Of the 17 serum samples and 14 rectal swab samples collected on the day of the diabetes diagnosis, none were positive for enteroviral RNA.
Viral sequence was obtained from 8 of 17 positive serum samples and from 33 of 45 positive rectal samples. The sequences were deposited in the GenBank sequence database under accession no. HM746666 – HM746706 (supplemental Table 3). Sequences are shown in supplemental Fig. 2 together with reference strain sequences listed in supplemental Table 3. Samples on which sequencing was not successful contained low concentrations of viral RNA. All samples but one clustered into enterovirus genogroup II, which contains, among others, the coxsackie B viruses ( 31 ) (supplemental Fig. 3). The sequence data indicated that viruses detected simultaneously in serum and rectal samples represented the same virus strain and only a single nucleotide substitution was once observed between such strains. Viruses detected in successive samples taken from the same individual represented different enterovirus strains. Thus, no evidence of viral persistence was found.
Progression to type 1 diabetes following enterovirus infections.
The progression to type 1 diabetes in the 17 intervals following detection of enteroviral RNA in serum was significantly more rapid (three type 1 diabetes cases diagnosed) than that in the 1,064 intervals following negative enteroviral RNA serum test (33 type 1 diabetes cases diagnosed; hazard ratio [HR]6.36) ( Table 2 ). Further adjustment for number of positive conventional islet autoantibodies did not alter the result ( Table 2 ). Because only three children were diagnosed in the interval after being positive for enteroviral RNA in serum, we employed a permutation test to make sure that the results of the standard inference based on Cox regression were valid. With 10,000 permutations, the (Monte Carlo) P value was 0.0075, thus confirming the highly significant result. After restricting the analysis to the 81% of samples with available data on ZnT8 autoantibodies, there was still a significant relation between serum enteroviral RNA and progression to type 1 diabetes (HR 6.21 [95% CI 1.82–21.2]), and this essentially was not affected by adjustment for ZnT8-autoantibody positivity in models without (8.50 [2.21–32.6]) or with (9.08 [2.30–35.8]) additional adjustment for the number of other islet autoantibodies.
Progression from islet autoimmunity to clinical type 1 diabetes in sample interval (median ∼4 months) following infection detected by enterovirus RNA in serum or rectal swab sample
Type of sample . | Person-years of follow-up . | Cases progressing to type 1 diabetes in interval . | Unadjusted HR (95% CI) . | HR (95% CI) adjusted for islet autoantibodies . |
---|---|---|---|---|
Serum | ||||
No enterovirus RNA in previous sample | 494 | 33 | 1.00 (ref.) | 1.00 (ref.) |
Enterovirus RNA in previous sample | 6.5 | 3 | 6.36 (1.89–21.4) | 7.02 (1.95–25.3) |
Rectal swab | ||||
No enterovirus RNA in previous sample | 537.1 | 32 | 1.00 (ref.) | 1.00 (ref.) |
Enterovirus RNA in previous sample | 21.2 | 1 | 0.93 (0.12–6.90) | 0.79 (0.10–5.92) |
Type of sample . | Person-years of follow-up . | Cases progressing to type 1 diabetes in interval . | Unadjusted HR (95% CI) . | HR (95% CI) adjusted for islet autoantibodies . |
---|---|---|---|---|
Serum | ||||
No enterovirus RNA in previous sample | 494 | 33 | 1.00 (ref.) | 1.00 (ref.) |
Enterovirus RNA in previous sample | 6.5 | 3 | 6.36 (1.89–21.4) | 7.02 (1.95–25.3) |
Rectal swab | ||||
No enterovirus RNA in previous sample | 537.1 | 32 | 1.00 (ref.) | 1.00 (ref.) |
Enterovirus RNA in previous sample | 21.2 | 1 | 0.93 (0.12–6.90) | 0.79 (0.10–5.92) |
Data are n unless otherwise indicated.
*Forty-one of 140 children in the study cohort progressed to type 1 diabetes during the period wherein collected samples were tested for enterovirus, of which serum enterovirus RNA results were available from the clinic visit preceding diagnosis in 36 (of which 3 were positive) and rectal swab enterovirus RNA was available in 33 (of which 1 was positive, and serum from the same visit was also positive for enterovirus RNA). Enterovirus exposure variables coded according to the rapid effect model described in research design and methods.
†Cox regression model: P = 0.003. Permutation test based on Cox regression model with 10,000 permutations of the enterovirus variable: P = 0.0075.
The three children who progressed to type 1 diabetes in the interval following a positive serum enteroviral RNA test all had typical characteristics of high risk for progression to type 1 diabetes. They had an early age at seroconversion for multiple islet autoantibodies and an affected sibling, and two of three carried the HLA DR3/4 genotype (supplemental Table 4). They also had a near-average interval length between clinic visits, and all were male and of non–Hispanic white ethnicity. Results were similar and remained statistically significant after restriction of the analysis to these respective subgroups (supplemental Table 5). Furthermore, the results were essentially unchanged after including four children who progressed to type 1 diabetes after being positive only once for islet autoantibodies (all four were negative for enteroviral RNA, adjusted HR 6.56 [95% CI 1.84–23.5]).
Presence of enteroviral RNA in rectal swabs did not predict progression to type 1 diabetes in the following sample interval (adjusted HR 0.79 [95% CI 0.10–5.92]) ( Table 2 ).
Analysis of progression to type 1 diabetes according to the cumulative number of enterovirus infections during follow-up, which allows for delayed effect, showed no significant relation with progression to type 1 diabetes for either serum or rectal swab enteroviral RNA or for serologically defined infections (supplemental Table 6). We also ran a Cox regression model simultaneously including variables modeling enterovirus according to the rapid effect model and the cumulative effect model. The results confirmed that the nonsignificant tendency toward an association for the cumulative effect variable was entirely due to the rapid effect, while the rapid effect of serum enteroviral RNA was unaltered and still significant (5.79 [1.23–27.3] for rapid effects model and 1.07 [0.37–3.11] for cumulative effect model).
There was also no relation between infections defined as increases in enterovirus antibodies and progression to type 1 diabetes according to the rapid effect model (supplemental Table 7). (Note that antibodies were only tested in the subset of samples collected during 1993–2004).
Finally, there were 19 children (61.3%) who progressed to type 1 diabetes among the 31 with one or more enteroviral RNA–positive serum or rectal swab samples compared with 31 (28.4%) among the 109 children in whom enteroviral RNA were not detected during follow-up ( P = 0.001). The proportion of visits where both serum and rectal swabs were positive for enteroviral RNA was higher among those who progressed to type 1 diabetes (6 of 425 prediagnostic visits [1.4%]) than among nonprogressors (2 of 603 visits [0.3%]), but this difference was not statistically significant.
To our knowledge, this is the first study to specifically assess the role of viral infections in the progression from islet autoimmunity to clinical type 1 diabetes in humans. We found that the rate of progression from islet autoimmunity to diabetes was significantly increased in sample intervals (of an average of 4 months) after the detection of enteroviral RNA in serum but not after detection of enteroviral RNA in rectal swab samples.
Strengths and limitations.
Given the amount of data available and many possible ways of analyzing data, we took great care to make all decisions a priori regarding algorithms for defining infections and methods of analysis. We used a formal cohort design and employed two main models (rapid effect and cumulative effect) to analyze two main indicators of enterovirus infections: enterovirus RNA in serum or in rectal swabs. Admittedly, our a priori–defined main exposure, presence of enterovirus RNA in either serum or rectal swabs, did not significantly predict progression to type 1 diabetes (supplemental Table 7). However, in preplanned subanalyses of serum and rectal swab enteroviral RNA examined separately, we found the presence of enterovirus RNA in serum to be a highly significant predictor of progression. Also, in the Finnish studies of enterovirus as a risk factor for islet autoimmunity, enteroviral RNA in serum samples have been more predictive than enteroviral RNA in stool samples ( 4 ). The number of children who progressed in sample intervals after the detection of viral RNA in serum was limited. However, rather than relying on standard inference alone, we confirmed the highly significant result using a permutation test, which is not susceptible to bias with small sample sizes. Furthermore, the result was consistent and remained significant in subgroups defined by characteristics of those who progressed to type 1 diabetes after enteroviral RNA was found in serum.
As a marker of islet autoimmunity, we used repeated presence of at least one islet autoantibody. This probably does not always reflect insulitis or activation of autoreactive T-cells, but autoantibodies are currently the best way of predicting type 1 diabetes in humans ( 1 ).
Interpretation.
Approximately 8% of the children progressing to type 1 diabetes had enteroviral RNA in their serum a few months prior to diagnosis. While our finding supports the hypothesis that infections resulting in enteroviral RNA in serum lead to a more rapid progression to clinical disease in some high-risk individuals, it may also suggest that enterovirus infection is a relatively uncommon cause of progression to type 1 diabetes. These observations may be explained by at least three potential scenarios.
First, we may be seeing only the tip of an iceberg because enterovirus is normally present in blood for only a few days during infection in immunocompetent hosts ( 5 , 32 ). Thus, the sampling intervals (median 4 months) are probably too wide to catch most of the causal infections, and enterovirus infections could turn out to be a major cause of progression from islet autoimmunity to diabetes. On the other hand, while viral shedding in feces rarely lasts more than 1 or 2 months ( 33 ), the prevalence of enteroviral RNA in rectal swab samples collected at ages <2.5 years in the current study was of a magnitude (8.7%) similar to that seen in other longitudinal studies with stool samples collected monthly from healthy children aged 3–28 months in Norway (11.5%) ( 33 ) and 3–22 months in Finland (6.0%) ( 34 ). To explain the lack of association between enteroviral RNA in rectal swabs, we may speculate that not all instances of gut infection are associated with a period with enteroviral RNA in the blood.
Second, enterovirus may establish low-grade persistent infection in children with islet autoimmunity, but the quantity of viral RNA in serum and feces may be below the detection limit in most such cases. Some studies have indicated presence of enterovirus in pancreatic tissue in a sizeable proportion of patients dying soon after onset of type 1 diabetes ( 35 , , – 38 ). Although the results varied depending on methodology and quality of specimens, detection of enterovirus in β-cells clearly strengthens the case for its role in the pathogenesis. In addition, a recent study suggests that the virus is present in the intestinal mucosa of diabetic patients ( 39 ). Enteroviral RNA was only detected at one time point in children diagnosed with type 1 diabetes during the sample interval following a positive serum enteroviral RNA test, which does not support the hypothesis of viral persistence. Sequence analysis did not give support for persistent infection because all sequences obtained from children with multiple infections were from different genotypes. Furthermore, it is notable that none of the samples collected at the day of diabetes diagnosis were positive for enteroviral RNA. This is consistent with a previous study of serum samples from Finland ( 5 ) but apparently inconsistent with the majority of studies of enterovirus RNA in plasma or serum taken from patients soon after diagnosis, which have found ∼30% of patients to be positive ( 40 , – 42 ). We have no explanation for this except a suggestion that an international laboratory standardization workshop could shed more light on these differences.
Third, enterovirus infection may be just one of many factors that can accelerate progression to diabetes, e.g., through nonspecific activation of autoreactive T-cells. Additional host and environmental factors are also likely to play a role. It is currently unclear whether certain enterovirus serotypes are more diabetogenic in humans than others. The most frequently implicated serotype, coxsackievirus B4 ( 43 ), was responsible for 2.4% of the enterovirus infectious episodes in the Norwegian study of healthy children ( 33 ). There may also be differences within serotypes because enteroviruses are known to mutate rapidly ( 4 , 32 ).
Another possibility that cannot be discarded is that progression to type 1 diabetes is enhanced because the viral infection induced insulin resistance sufficient to precipitate clinical disease. Nonspecific febrile illness or other infectious symptoms in a period prior to diagnosis seems to be quite commonly reported, but few studies have been able to obtain comparable data in age-matched controls ( 44 ) and the large majority of enterovirus infections are asymptomatic ( 32 ). Furthermore, while biopsy studies and previous cross-sectional or retrospective studies of enterovirus infections in patients with type 1 diabetes cannot exclude the possibility that the disease influenced the risk of infection, our longitudinal design allowed us to draw stronger inference in this regard. The fact that none of the children who were tested on the day of diagnosis were positive for enteroviral RNA, including those who were enterovirus positive in the interval before diagnosis, shows that reverse causation was unlikely.
A number of potential mechanisms for how viral infections may induce or accelerate autoimmune diabetes have been proposed, mostly based on animal models or in vitro studies ( 43 , 45 ). Mechanisms in humans are likely to be complex, but may initially involve, for example, activation of the innate immune system, secretion of interferon-α, and perhaps upregulation of major histocompatibility complex molecules on β-cells ( 46 ). Results from animal models cannot automatically be generalized to humans, but studies in strains of NOD mice have indicated a requirement for preceding β-cell damage and release of β-cell antigens taken up by antigen-presenting cells ( 13 , 47 ), as previously reviewed ( 43 ). The “fertile field hypothesis” proposes that different viruses may increase the risk of diabetes in susceptible time windows after an infection, while outside this window a similar viral infection would be resolved with no further consequences for the host ( 3 ).
Future studies and final conclusion.
Despite the huge undertaking of screening and prospectively following a large number of children for several years, the number of end points was still limited and independent replication in future studies would strengthen the results. Children who progressed to type 1 diabetes immediately after detection of enterovirus RNA all had clinical characteristics consistent with high risk of progression such as early development of multiple islet autoantibodies. Future studies could investigate further the potential role of additional host and viral factors in this process. Prospective studies are challenging, and up to now such studies have mainly focused on the initiation of autoimmunity as the end point ( 7 , – 9 , 26 ), with mixed results. The Environmental Determinants of Diabetes in the Young (TEDDY) study ( 48 ) has the potential to provide answers concerning the role of enterovirus and progression to type 1 diabetes with greater power and avoiding some of the limitations of the study presented here.
In conclusion, the rate of progression from islet autoimmunity to type 1 diabetes was significantly increased in the approximately 4-month interval following detection of enteroviral RNA in serum.
The costs of publication of this article were defrayed in part by the payment of page charges. This article must therefore be hereby marked “advertisement” in accordance with 18 U.S.C. Section 1734 solely to indicate this fact.
This study was supported by National Institute of Diabetes and Digestive and Kidney Diseases grants DK-32493, DK-32083, and DK-50979; Diabetes Endocrinology Research Center Clinical Investigation and Bioinformatics Core P30 DK 57516; and the Children's Diabetes Foundation. L.C.S. was supported in part by a grant from the National Research Council of Norway (148359/330). Virus analyses in the laboratory of H.H. were partly supported by a grant from the Academy of Finland.
H.H. is a shareholder (<5%) in Vactech LTD, Tampere, Finland, a company that develops vaccines against picornaviruses. No other potential conflicts of interest relevant to this article were reported.
L.C.S. planned the present study with assistance from K.J.B., designed the analysis strategy, did the statistical analyses, and wrote the manuscript with input from all authors. H.H. and S.O. were responsible for the enterovirus testing and enteroviral sequence analysis. K.B. was responsible for managing and preparing the databases. G.K. was responsible for the clinical evaluation and diagnosis of type 1 diabetes. J.C.H. was responsible for measuring the ZnT8 autoantibodies. H.A.E. was responsible for the HLA genotyping. G.S.E. was responsible for measuring the anti-insulin, -GAD, and –IA-2 autoantibodies. M.R. was the principal investigator and developed the general protocol for the DAISY study with input from J.M.N., G.K., H.A.E., and G.S.E.
The authors thank the DAISY and Barbara Davis Center staff for their help, Liping Yu at the Barbara Davis Center for running the autoantibody assays, and Dory Bugawan at Roche Molecular Systems for help with the HLA genotyping.

Supplementary data
Email alerts.
- Online ISSN 1939-327X
- Print ISSN 0012-1797
- Diabetes Care
- Clinical Diabetes
- Diabetes Spectrum
- Standards of Medical Care in Diabetes
- Scientific Sessions Abstracts
- BMJ Open Diabetes Research & Care
- ShopDiabetes.org
- ADA Professional Books
Clinical Compendia
- Clinical Compendia Home
- Latest News
- DiabetesPro SmartBrief
- Special Collections
- DiabetesPro®
- Diabetes Food Hub™
- Insulin Affordability
- Know Diabetes By Heart™
- About the ADA
- Journal Policies
- For Reviewers
- Advertising in ADA Journals
- Reprints and Permission for Reuse
- Copyright Notice/Public Access Policy
- ADA Professional Membership
- ADA Member Directory
- Diabetes.org
- X (Twitter)
- Cookie Policy
- Accessibility
- Terms & Conditions
- Get Adobe Acrobat Reader
- © Copyright American Diabetes Association
This Feature Is Available To Subscribers Only
Sign In or Create an Account

An official website of the United States government
The .gov means it’s official. Federal government websites often end in .gov or .mil. Before sharing sensitive information, make sure you’re on a federal government site.
The site is secure. The https:// ensures that you are connecting to the official website and that any information you provide is encrypted and transmitted securely.
- Publications
- Account settings
The PMC website is updating on October 15, 2024. Learn More or Try it out now .
- Advanced Search
- Journal List
- HHS Author Manuscripts

Pancreatic islet reserve in type 1 diabetes
Anneliese j. s. flatt.
1 Translational and Clinical Research Institute, Newcastle University, Newcastle upon Tyne, UK.
2 Institute of Transplantation, Freeman Hospital, Newcastle upon Tyne, UK.
Carla J. Greenbaum
3 Diabetes Program and Center for Interventional Immunology, Benaroya Research Institute, Seattle, Washington.
James A. M. Shaw
Michael r. rickels.
4 Division of Endocrinology, Diabetes and Metabolism, Department of Medicine, Hospital of the University of Pennsylvania, Philadelphia, Pennsylvania.
5 Institute for Diabetes, Obesity and Metabolism, University of Pennsylvania Perelman School of Medicine, Philadelphia, Pennsylvania
Type 1 diabetes (T1D) is a chronic autoimmune disease characterized by pancreatic islet β cell loss and dysfunction resulting in insulin deficiency and hyperglycemia. During a presymptomatic phase of established β cell autoimmunity, β cell loss may first be evident through assessment of β cell secretory capacity, a measure of functional β cell mass. Reduction in pancreatic islet β cell reserve eventually manifests as impaired first-phase insulin response to glucose and abnormal glucose tolerance, which progresses until the functional capacity for β cell secretion can no longer meet the demand for insulin to control glycemia. A functional β cell mass of ~25% of normal may be required to avoid symptomatic T1D but is already associated with dysregulated glucagon secretion. With symptomatic T1D, stimulated C-peptide levels >0.60 ng/mL (0.200 pmol/mL) indicate the presence of clinically meaningful residual β cell function for contributing to glycemic control, although even higher residual C-peptide appears necessary for evidencing glucose-dependent islet β and α cell function that may contribute to maintaining (near)normal glycemia.β cell replacement by islet transplantation can restore a physiologic reserve capacity for insulin secretion, confirming thresholds for functional β cell mass required for independence from insulin therapy.
Introduction
Type 1 diabetes (T1D) is a disease of insulin deficiency characterized by autoimmune destruction of pancreatic islet β cells. Now, 100 years since the discovery of insulin in 1921–1922, the clinical course of T1D has been transformed. However, insulin therapy by injection or subcutaneous infusion informed by ongoing glucose monitoring remains an unremitting daily challenge, with a continued risk of complications secondary to chronic high glucose exposure and dangerous acute episodes of hypoglycemia. 1 – 5
T1D initially follows a subclinical course and presents at the point when residual β cell functional capacity can no longer meet the demand required for the maintenance of normal glucose homeostasis. 6 The subclinical course of T1D described as early as the 1980s helped to establish the Eisenbarth model of a chronic autoimmune disorder targeting the insulin-producing β cell initiated by an interaction of genetic and environmental factors and progressing at a variable rate until insufficient reserve capacity for insulin secretion results in the clinical presentation of diabetes. 7 – 11 As family history is associated with an increased risk of T1D, with a 1-in-20 lifetime risk in first-degree relatives compared with a 1-in-300 lifetime risk for the general U.S. population, 12 family studies have been invaluable in advancing the understanding of diabetes pathogenesis, including its subclinical course to better inform pre-clinical staging. 11 , 13 , 14 The genetic predisposition is largely mediated through human leukocyte antigen (HLA) genotype DR3/DR4-DQ8 in addition to non-HLA–related genes, 15 – 18 which in combination with one or more still undefined environmental factors can lead to seroconversion through the development of autoantibodies against β cell–specific antigens. These include glutamic acid decarboxylase 65 (GAD-65), the tyrosine phosphatase–related islet antigen 2 (IA-2), insulin (IAA), and zinc transporter 8 (ZnT8), with their autoantibodies representing markers of β cell autoimmunity.
Once autoimmunity is established, subclinical T1D can progress through a presymptomatic phase that can be identified clinically using biomarkers of β cell autoimmunity and dysglycemia before the development of overt, symptomatic diabetes ( Table 1 and Ref. 19 ). During subclinical disease progression, the pancreatic islet reserve capacity for insulin secretion declines as increasing numbers of β cells are lost to autoimmune destruction. This reserve capacity for secretion normally maintains glucose homeostasis during periods of growth and times of stress, and as it becomes limited results in the appearance of glucose intolerance, and once exhausted, the development of overt diabetes. Thus, clinically, T1D may be considered a disease from the time autoimmunity is established before devel oping glucose intolerance (stage 1), progressing to glucose intolerance (stage 2), and leading to typical signs and symptoms of diabetes (stage 3). 19
Stages in the pathogenesis of type 1 diabetes 19
Nomeclature | Prestage 1 | Stage 1 | Stage 2 | Stage 3 |
---|---|---|---|---|
Natural history | Genetic and environmental susceptibility | Presymptomatic | Presymptomatic | Symptomatic |
β cell autoimmunity | 0–1 autoantibodies | 2 or more autoantibodies | 2 or more autoantibodies | 2 or more autoantibodies |
Glucose tolerance and glycemia | Normal, normoglycemia | Normal, normoglycemia | Impaired, dysglycemia | Diabetic, dysglycemia |
First-phase insulin response | Normal | Normal to mild impairment | Impaired | Absent |
β cell secretory capacity | Normal | Normal to moderate impairment | Variable impairment | Markedly impaired to absent |
One goal of staging presymptomatic T1D is to enable early immune intervention strategies aimed at preventing or ameliorating symptomatic diabetes through the preservation of sufficient pancreatic functional β cell mass to maintain (near)normoglycemia. 20 Preservation of endogenous β cell function after clinical diagnosis is also important in facilitating optimal metabolic control. The objective of this review is to examine the physiological and clinical correlates of pancreatic islet reserve at each stage of T1D in order to aid understanding of pancreatic islet physiology informing interventions to maintain or restore a functional β cell mass.
Islet reserve and function in health
Pancreatic islets are distributed throughout the pancreatic acinar/exocrine tissue and account for 2–3% of pancreatic mass. Human islets are composed predominantly of insulin-producing β cells and glucagon-producing α cells with the remaining 10% comprising somatostatin-producing δ cells, pancreatic polypeptide producing PP cells, and ghrelin-producing ɛ cells. 21 Nervous system innervation, β cell electrical activity, and intraislet paracrine signaling support glucose-dependent insulin and glucagon secretion. 22 Insulin secretion is pulsatile in nature, with pulses occurring every ~5–6 min and (in the nondiabetic state) increasing in amplitude in response to glucose. 23 In healthy individuals, insulin secretion varies by several orders of magnitude to meet the demand for control of glucose homeostasis. This is accomplished both by biphasic kinetics of insulin secretion and recruitment of additional β cells to an activated state, which provides the reserve capacity for secretion. The first-phase secretion involves rapid exocytosis of insulin granules from a readily releasable pool. Under conditions of a sustained glucose stimulus, this first phase is followed by a subsequent second, more prolonged phase of insulin release from the mobilization of granules within the reserve pool, comprising 95% of β cell insulin granules. 24 Heterogeneity of β cell functional states has long been appreciated, 25 and more recently, evidence has emerged supporting a subpopulation (<10% of β cells) that functions as a central hub, coordinating integrated β cell response to stimuli. 26 Thus, normal insulin secretion appears dependent on coordinated β cell function from intact islets.
Normal insulin secretion is also dependent on the presence of sufficient reserve capacity of functional β cell mass. Functional β cell mass is best estimated from the β cell secretory capacity derived from glucose-potentiation of insulin or C-peptide release in response to injection of a nonglucose secretagogue, such as arginine or glucagon. 27 Glucose potentiation creates the condition for second-phase insulin secretion, whereby mobilization of secretory granules from the reserve pool of an increased number of activated β cells results in augmented acute insulin release in response to membrane depolarization induced by the nonglucose secretagogue. Increased islet blood flow and islet oxygenation has been demonstrated in animal models of partial pancreatectomy and gestation, suggesting the recruitment of a functional reserve capacity in response to increasing β cell demand for secretion occurs without an increase in β cell mass. 28 , 29 Nonetheless, the recruitment of senescent β cells and generation of new β cells from neogenesis or self-duplication of existing β cells may also contribute to increasing β cell secretory capacity for increased demand, as shown in rodent models. 30 The risk of glucose intolerance secondary to a marked reduction in pancreatic mass is evidenced in healthy hemipancreatectomy donors in whom a residual 40% β cell secretory capacity was associated with reduced first-phase insulin secretion in response to glucose, as well as the development of glucose intolerance, and long-term risk of progression to diabetes, especially in association with weight gain. 31 , 32 Another study involving individuals with exocrine pancreatic disease undergoing pancreatic surgery showed that diabetes was associated with a reduction in relative β cell area (assessed from sections of resected pancreatic tissue) to 30–40% of that in nondiabetic individuals. 33 Indeed, both human and animal models suggest an islet mass of ~50% may be the threshold for maintaining sufficient β cell functional reserve for normal glucose control. 34 – 36 Thus, while the range is variable among individuals, 37 , 38 in general, with less than 50% of normal β cell secretory capacity, there may be insufficient islet reserve (including loss of first-phase insulin secretion) to meet demand. Other work reports that even mild hyperglycemia following 90% partial pancreatectomy in animal models is associated with changes in β cell–specific gene expression profiles, 39 supporting a role for β cell dysfunction in addition to absolute β cell mass loss in contributing to insulin secretory defects with potential consequences for long-term β cell health.
There exists reciprocal glucose-dependent regulation of β cell insulin secretion and α cell glucagon secretion in the normal pancreatic islet, such that increasing glucose potentiates insulin secretion and inhibits glucagon secretion in order to avoid hyperglycemia, while decreasing glucose inhibits insulin secretion and activates glucagon secretion in order to avoid hypoglycemia. 22 Hemipancreatectomy resulting in 40% of normal β cell secretory capacity is associated with 35% of normal acute glucagon response to arginine with and without glucose inhibition, 31 in keeping with equivalent reductions in both pancreatic islet β and α cell mass. Importantly, despite reduced pancreatic islet mass, the α cell glucagon secretion in response to hypoglycemia is seemingly normal, 40 and it is proposed that the remaining intact islets exhibit normal paracrine activation of glucagon secretion by the intraislet decrement in insulin secretion that occurs with the development and in defense of hypoglycemia, 41 leading to physiological secretion despite a substantial decrease in α cell mass.
Islet reserve and function at early disease stages, before clinical diagnosis
Longitudinal studies continued over several decades of follow-up have confirmed that the presence of β cell autoimmunity, as evidenced by seroconversion of two or more islet autoantibodies, almost inevitably heralds progression to the clinical diagnosis of T1D. 42 – 44 While considered prestage 1 disease, autoimmunity manifested by the presence of only a single autoantibody also confers risk with ~20% developing multiple antibodies within 5 years and ~15% progressing to clinical diabetes despite remaining with single autoantibody positivity. 45 The stages of the diabetes framework describe the progression of disease by changes in glycemic status and has proved to be useful for refining inclusion and outcome criteria for clinical trials aiming to slow or delay disease progression. T1D is, however, primarily a disease of β cell dysfunction and loss with the secondary consequence of glucose dysregulation. β cell secretory abnormalities are present long before clinical diagnosis; 46 but interestingly, often not well correlated with either the number of autoantibodies or glucose tolerance.
For example, Vandemeulebroucke et al . assessed functional β cell mass using a hyperglycemic clamp with glucagon stimulation to measure β cell secretory capacity in 17 IA-2 antibody–positive first-degree relatives followed over 5 years during which 50% were predicted to develop clinical diabetes. 47 Ten of these individuals did not develop clinical diabetes over the 5-year follow-up and had entirely normal oral glucose tolerance and β cell secretory capacity at baseline, not different from a control group with genetic risk for T1D without antibodies. 47 The seven individuals who did progress to clinical diabetes from 3 to 63 months after baseline study had oral glucose tolerance that was initially similar to that in the group of nonprogressors and controls, but β cell secretory capacity that was <50% of normal, indicating the presence of a substantial reduction in functional β cell mass. 47 This small study suggests that the presence of two or more islet autoantibodies does not, in itself, indicate a reduction in pancreatic islet reserve, and moreover, that identification of a reduced β cell secretory capacity appears to be more sensitive than assessment of oral glucose tolerance (OGTT) for detecting those likely to progress more rapidly to overt diabetes.
β cell function in autoantibody-positive individuals is most often assessed from insulin or C-peptide levels obtained during an oral glucose tolerance test (OGTT) or from the first-phase insulin response (FPIR) during an intravenous glucose tolerance test (IVGTT). Decreased insulin secretion in individuals with early-stage T1D confers an increased risk of eventual clinical disease, 48 but strikingly, low FPIR is only moderately associated with glucose intolerance in cross-sectional studies ( r 2 = 0.114). 49 However, a separate study in type 2 diabetes (T2D) found that once fasting glucose exceeds 115 mg/dL, FPIR is absent, 50 likely signifying the exhaustion of a reserve capacity for insulin secretion at that time. Studies by Hao et al . have shown significant variation in insulin secretory reserve/functional β cell mass, assessed by the acute insulin response to glucose-potentiated arginine stimulation, in antibody-positive individuals with reduced β cell function, assessed by the FPIR. 51 It has previously been shown that the insulin response to glucose is lost earlier than the response to arginine in settings of a reduced β cell mass, including both presymptomatic T1D 52 and recipients of islet transplantation, 53 groups characterized by a marked reduction in functional β cell mass, where acute insulin responses to arginine are maintained despite marked impairment of or loss of FPIR. Evidence for differences in various surrogate measures of functional β cell mass following oral or intravenous stimulatory challenges further supports underlying heterogeneity in T1D pathogenesis. 51
The rate of progression from autoimmunity to clinical diabetes is variable. 54 β cell glucose sensitivity, measured by the slope of insulin secretion in response to increasing plasma glucose, may be impaired several years before diagnosis and so might represent an early sign of β cell dysfunction nearing a critical threshold of insulin deficiency beyond which clinical diabetes ensues. 55 The Diabetes Prevention Trial-Type 1 risk score (DPTRS) can assess risk for future diabetes in autoantibody-positive individuals using age, body mass index, fasting C-peptide, and the 30-, 60-, 90-, and 120-min C-peptide and glucose values during a 2-h OGTT. 56 Using only the 60-min C-peptide and glucose (DPTRS60) was recently shown to predict diabetes development with similar accuracy. 57 Importantly, a 1-h OGTT glucose ≥155 mg/dL (8.6 mmol/L) had slightly greater receiver operating characteristics (sensitivity versus 1–specificity) than the standard 2-h OGTT glucose ≥140 mg/dL (7.8 mmol/L) for predicting future diabetes development. 57 The potentially greater utility of the 1-h OGTT glucose to reflect the early loss of pancreatic islet secretory reserve in T1D is similar to that observed in individuals with pancreatic insufficient cystic fibrosis, where a 1-h OGTT glucose ≥155 mg/dL (8.6 mmol/L) is associated with an already marked reduction in β cell secretory capacity. 58
Follow-up of a cohort of individuals with autoantibody positivity and relatives with T1D by 6-monthly OGTT toward the onset of diabetes in the Diabetes Prevention Trial-Type 1 showed a progressive decline in glucose tolerance over the 2-year period preceding diagnosis, with a more rapid decline in stimulated C-peptide occurring in the last 6 months. 59 More recently, Bogun et al . demonstrated that this accelerated rate of fall in insulin secretion persists during and beyond the peridiagnostic period. 60 Whether this accelerated decline in β cell function prior and through the peridiagnostic period is related to ongoing immunologic destruction and/or metabolic exhaustion of β cells is unknown.
Some individuals have been reported to have disproportionately increased secretion of proinsulin relative to insulin or C-peptide during disease progression, as assessed by the elevated fasting proinsulin-to-C-peptide ratio before diagnosis. 61 Similar to observations in T2D, impaired proinsulin processing before secretion is suggestive of β cell endoplasmic reticulum stress; however, the relationship of the abnormal proinsulin-to-C-peptide ratios to either autoimmunity or glucose tolerance remains unclear. It is likely that both the dysfunction of β cells in intact islets and the loss of islet β cells due to chronic β cell destruction contribute to disease progression toward diabetes. Whether individuals with a greater dysfunctional component underlying reduced insulin secretion but relatively intact β cell secretory capacity indicating preserved β cell mass may be more likely to respond to disease-modifying therapy requires further investigation. 51
Islet reserve and function after clinical diagnosis
Stage 3 T1D is defined by the onset of clinical disease through the presence of signs and symptoms of hyperglycemia or when asymptomatic by crossing the diagnostic glucose thresholds established by the World Health Organization/American Diabetes Association. The prospective monitoring of individuals with presymptomatic disease can enable early detection of diabetes through the confirmation of fasting glucose ≥126 mg/dL (≥7.0 mmol/L); 2-h OGTT glucose ≥200 mg/dL (≥11.1 mmol/L); and/or an HbA 1c ≥6.5% (48 mmol/mol) before the onset of symptoms. ≥ Individuals with early, but still asymptomatic, stage 3 T1D defined by a 2-h OGTT glucose ≥200 mg/dL (≥ 11.1 mmol/L) who are able to maintain nondiabetic levels of fasting glucose and HbA1c already exhibit markedly impaired insulin secretion in response to oral and intravenous glucose, yet preserved FPIR to the nonglucose β cell secretagogue arginine. 62 This may be explained by the potentiation of insulin release to nonglucose secretagogues by increasing fasting glucose concentration. The reduction in overall β cell secretory capacity is, however, observed by reduced insulin release in response to arginine potentiated by submaximal (AIR pot ) and maximal (AIR max ) hyperglycemic clamp conditions. Indeed, glucose-potentiated arginine testing demonstrated that the β cell secretory capacity in this cohort of individuals with asymptomatic T1D was only 25% of that in a normal control group. 62
In another study of new-onset T1D, the median β cell secretory capacity of 80 individuals was again 25% of that measured in 20 healthy, agematched control subjects using glucose potentiation of glucagon-induced insulin release. 63 Here, individuals with β cell secretory capacity at or above the 25th percentile were more likely to experience the preservation of β cell function and a reduction in insulin requirements 18 months after receiving a 6-day course of anti-CD3 antibody therapy. 63 Thus, a functional β cell mass of at least 25% normal may be required to avoid symptomatic T1D and may also represent a threshold that allows for the easier demonstration of protection of residual β cell function. However, it is unknown whether individuals with lower pancreatic islet reserve at clinical diagnosis have more severe and potentially treatment-resistant autoimmunity or simply greater and potentially less reversible loss of functional β cell mass. Interestingly, at a β cell secretory capacity <25% of normal adults, insulin release to nonglucose secretagogues is already maximal in the fasted state in the presence of elevated glucose concentrations, such that further glucose potentiation of insulin release to arginine under hyperglycemic clamp conditions is no longer possible ( Fig. 1 ).

β cell secretory capacity data from an individual with adult-onset T1D, initially diagnosed with T2D and at the time of glucose-potentiated arginine testing before initiating insulin therapy. Under fasting conditions, the acute insulin (A) or C-peptide (B) response to arginine appear “normal” (although not when considering the fasting glucose was elevated at 162.5 mg/dL (not shown)), and further elevation of the glucose cannot further potentiate insulin release as the entire reserve capacity for secretion (~15% of normal) is already engaged under the fasting condition. The normal data are 95% CI based on 28 nondiabetic individuals.
At the point when pancreatic islet reserve for β cell insulin secretion is compromised in T1D, marked abnormalities in α cell regulation of glucagon secretion are also evident. Metabolic testing through OGTT and IVGTT at the earliest identification of stage 3 T1D reveals impaired suppression of glucagon secretion in response to hyperglycemia. 62 This impaired regulation of glucagon secretion in T1D may be explained by the loss of paracrine effects of β cells on α cell function in islets, where β cells are destroyed or no longer functional due to β cell autoimmunity. The absence of suppression of glucagon during oral glucose or mixed-meal tolerance testing (MMTT) likely contributes to postprandial hyperglycemia in early T1D. 62 , 64
In addition, glucagon response to hypoglycemia is already markedly impaired at diabetes onset. 65 , 66 Proposed underlying mechanisms include altered islet innervation, abnormal α cell glucose sensing, and reduced intraislet paracrine signaling, whereby β cell deficiency leads to an insufficient decrement of insulin in response to hypoglycemia to act as a paracrine trigger for α cell glucagon release (reviewed by Rickels 67 ). Whatever the mechanism, an impaired glucagon response to hypoglycemia is observed uniformly in recent-onset diabetes regardless of the level of residual C-peptide. 66 , 68
Brissova et al . have provided cellular evidence for an inherent α cell defect in T1D, with the reduction in transcription factors associated with α cell identity and reduced expression of exocytosis and signaling-related proteins demonstrated in islets isolated from individuals with T1D. 69 Isolated islets showed the preservation of glucose-stimulated insulin secretion from the low number of β cells present; however, glucagon release to low glucose incubation was deficient despite the observation of an increased number of α cells in islets from the individuals with T1D compared with controls. Although a relative increase in α to β cells is anticipated due to β cell loss, this observation of increased numbers but the impaired function of α cells suggests an additional role for islet cell dedifferentiation in diabetes pathogenesis, a finding that is supported by pathology studies in T2D. 70 Similar data available from the Human Pancreas Analysis Program ( https://hpap.pmacs.upenn.edu ) demonstrate seemingly appropriate dynamics of insulin secretion in response to glucose, albeit at the markedly reduced magnitude, and paradoxically increased glucagon secretion ( Fig. 2A ). Interestingly, the transplantation of islets from individuals with T1D into a normoglycemic nonautoimmune environment led to partial restoration of gene expression changes toward a normal α cell phenotype. 69

(A) The perifusion of islets isolated from a deceased donor with a 5-year duration of T1D. Insulin release is appropriate in response to change from low glucose (G; 3 mM) to high glucose (G; 16.7 mM), and to phosphodiesterase inhibition with 3-isobutyl-1-methylxanthine (IBMX, 0.1 mM) and membrane depolarization with KCl, albeit at the markedly reduced magnitude. Glucagon release is appropriate in response to amino acid mixture (AAM, 4.0 mM), IBMX, and KCl, but paradoxically increased (failed suppression) in response to change from low to high glucose. (B) Imaging mass cytometry of pancreatic sections from the same deceased donor demonstrates the presence of few islets with normal numbers of β cells (green) and more representative islets containing α (red) and δ (blue) but no β cells. Data are available at https://hpap.pmacs.upenn.edu .
In vitro studies have demonstrated the down-regulation of key β cell transcription factors and changes in β cell phenotype associated with hyperglycemia, which, to some extent, may be reversible with normalization of glycemia. 39 However, the extent to which β cell senescence, dedifferentiation, and transdifferentiation are implicated in β cell dysfunction and loss in human T1D remains unclear. 39 Intensive insulin use at diagnosis has been associated with a period of preservation of C-peptide production 71 , 72 with the fasting euglycemia attained from exogenous insulin therapy, perhaps contributing to β cell “rest” and possible protection from the phenotypic and functional changes associated with β cell stress. Similar “remission” is seen with aggressive glycemic control in those with T2D. However, the failure of a randomized trial to confirm the preservation of functional β cell mass with intensive insulin therapy in recently diagnosed T1D patients, 73 as well as the failure of parenteral insulin to delay disease progression in the early stages of disease, 74 suggests that the benefit of insulin therapy on the preservation of secretion is only manifest with marked initial hyperglycemia, supporting the hypothesis that glucotoxicity plays a role in β cell dysfunction with a potentially reversible component.
Islet reserve and function in established clinical disease
Functional β cell mass continues to decline following a diagnosis such that the majority of individuals have a stimulated C-peptide <0.60 ng/mL (0.200 pmol/mL) within 5 years of symptomatic diagnosis. 75 This decline is dependent on the age at symptomatic disease onset, with individuals presenting as adults >18 years old tending to maintain residual β cell function longer than those presenting as children. 76 In the Diabetes Control and Complications Trial (DCCT), those individuals with a mixed-meal stimulated C-peptide >0.60 ng/mL (0.200 pmol/mL) within 5 years of symptomatic diagnosis had a lower incidence of retinopathy and nephropathy and a decreased prevalence of severe hypoglycemia than those with lower amounts of residual C-peptide detection regardless of treatment intensity. 77 , 78 These results fairly conclusively support that preservation of residual β cell function characterized by stimulated C-peptide >0.60 ng/mL (0.200 pmol/mL) is clinically meaningful and supports immunotherapy approaches toward the preservation of functional β cell mass (reviewed by Lord and Greenbaum 79 ). A more recent analysis of the DCCT suggests that any level of stimulated C-peptide above the detection threshold of 0.09 ng/mL (0.03 pmol/mL) used at trial entry may be associated with better clinical outcomes. 80
The development of highly sensitive C-peptide assays led to the detection of very low levels of C-peptide (that would have been “undetectable” in the DCCT) in the majority of individuals over the first 10 years of symptomatic T1D and in a declining minority of individuals more than a decade after clinical diagnosis. 81 – 83 These observations are consistent with Yu et al ., who identified few scattered insulin-positive cells in all individuals with T1D at autopsy. 84 Follow-up of individuals at least 5 years after diagnosis showed that circulating proinsulin may persist even in the absence of detectable C-peptide. Whether this is caused by ongoing (immunologically or metabolically mediated) β cell stress or represents an irreversible shift in β cell phenotype precluding normal proinsulin processing remains unclear. 85 , 86 Assessment of type 1 donor islets from pancreatic sections obtained from the Network for Pancreatic Organ Donors with Diabetes demonstrated the reduced expression of the proinsulin processing enzymes, prohormone convertase 1/3, and carboxypeptidase, compared with nondiabetic controls, which may account for the elevated proinsulin:C-peptide ratios in individuals with the lowest detectable β cell peptides. 87
Sustained C-peptide, >0.60 ng/mL (0.200 pmol/mL) in a random sample, has been observed in members of the Joslin Medalist group despite more than 50 years of insulin-dependent diabetes. In this cohort, sustained C-peptide was associated with a later age of diagnosis and the presence of DR3 risk alleles. 88 Postmortem examination of pancreata from nine Medalists showed significant numbers of persisting insulin-positive cells scattered as single extra-islet cells or as single cells within some islets or many cells in some islets in several lobes among many insulin-negative islets despite the long duration of diabetes. 88 Insulin-positive islets were more likely to be present in later-onset diabetes. However, even Medalists without detectable C-peptide on MMTT could have evidence of residual insulin-positive β cells present as single cells within islets. 84 , 88 Nevertheless, the number of residual insulin-positive cells correlated with the preceding MMTT C-peptide response such that those who experienced a physiologically significant doubling of C-peptide on stimulation had considerably more insulin-positive cells found within islets of certain lobules of the pancreas. 88 This finding suggests that physiological inducible insulin secretion may depend on the presence of residual β cells in intact islets rather than occur from scattered insulin-positive cells ( Fig. 2B ).
A subsequent study of 68 individuals in the Joslin Medalist cohort by Yu et al . identified three patterns of residual insulin staining, including scattered insulin-positive cells present in all samples; few insulin-positive cells within some islets in ~87%; and many insulin-positive cells in some islets within certain lobes of tissue present in ~20% of samples. 84 Prospective follow-up of C-peptide and autoantibody status of Medalists over 4 years showed low levels of detectable C-peptide (random sample >0.05 ng/mL (0.017 pmol/mL)) were present in ~32% of individuals. Detectable C-peptide was associated with IA2 autoantibody positivity, with GAD positivity more commonly observed in those with undetectable C-peptide. However, antibody titers and indeed C-peptide levels fluctuated over follow-up, suggestive of possible episodic autoimmune aggravation alternating with at least minimal recovery of some β cell function despite long-standing disease duration. 84 Preserved lobes containing insulin-positive islets were associated with greater C-peptide, lower HbA 1c , and older age at diagnosis compared with individuals with only residual scattered insulin-positive cells (within and outside islets). These findings further suggest the importance of β cell contact/islet microenvironment in the maintenance of physiological insulin secretion. 84
Rickels et al . assessed the physiological significance of varying levels of residual C-peptide in individuals with established T1D stratified by MMTT peak C-peptide as negative (<0.02 ng/mL (0.007 pmol/mL)), low (0.05–0.60 ng/mL (0.017–0.200 pmol/mL)), intermediate (0.60–1.20 ng/mL(0.200–0.400 pmol/mL)), and high (>1.20 ng/mL (>0.400 pmol/mL)). 89 Functional β cell mass, assessed by the acute C-peptide response to glucose-potentiated arginine, was shown to be highly associated with MMTT peak C-peptide, 89 a relationship also seen with the MMTT C-peptide area-under-the-curve ( Fig. 3 ). Despite this continuous association between MMTT peak C-peptide and β cell secretory capacity as a measure of functional β cell mass, only the high C-peptide group showed β cell responsivity to glucose during a hyperglycemic clamp before the injection of arginine ( Fig. 4A ) and α cell responsivity to insulin-induced hypoglycemia during a hyperinsulinemic hypoglycemic clamp ( Fig. 4B ). The increased glucagon response to hypoglycemia observed in the high C-peptide group may depend on functional β cell suppression within intact islets activating neighboring α cells. However, even with high residual C-peptide, β cell secretion of insulin in response to meal or arginine stimulation does not suppress α cell glucagon secretion. 89 This dysregulation of α cell function may be explained by the failure of glucagon suppression from the majority of diseased islets that do not contain β cells.

Relationship between β cell secretory capacity measured from the acute C-peptide response to glucose-potentiated arginine and the mixed-meal tolerance test (MMTT) area-under-the-curve (AUC) C-peptide response over 120 min in individuals with MMTT peak C-peptide defined as: low, 0.05–0.60 ng/mL (0.17–0.20 pmol/mL); intermediate, >0.60–1.20 ng/mL (0.20–0.40 pmol/mL); or high, >1.20 ng/mL(>0.40 pmol/mL). Data are from Ref. 89 .

(A) β cell response to an increase in glucose during a hyperglycemic clamp, and (B) α cell response to insulin-induced hypoglycemia during a hypoglycemic clamp in individuals with T1D by peak C-peptide during a mixed-meal tolerance test (negative, <0.02 ng/mL; low, 0.05–0.60 ng/mL; intermediate, >0.60–1.20 ng/mL; and high, >1.20 ng/mL). An increase in C-peptide in response to hyperglycemia and an increase in glucagon in response to hypoglycemia were observed only in the group with the highest residual C-peptide production that may represent a minimal functional islet reserve. Data are from Ref. 89 .
Importantly, individuals with high MMTT peak C-peptide who have evidence of preserved islet β and α cell responsivity to glucose also exhibit lower mean glucose and greater time in range on continuous glucose monitoring ( Fig. 5A ). 89 Consistent with these findings, a study investigating the glycemic effects of 45 min of aerobic exercise in T1D found that individuals with higher MMTT peak C-peptide (>0.60 ng/mL (0.200 pmol/mL)) spent more time in range on continuous glucose monitoring during the subsequent 24 h than those with low or undetectable C-peptide, and while not statistically significant given the small sample size, spent twofold less time with hypoglycemia during the postexercise period. 90 Thus, improved glycemic control in individuals with high residual C-peptide may be explained by a valuable functional reserve of preserved β and α cell responsivity to glucose, which can refine the effects of exogenous insulin, but which is less apparent with lower residual C-peptide production. Nevertheless, the Scottish Diabetes Research Network Type 1 Bioresource following over 6000 individuals with T1D has shown that after adjusting for diabetes duration, even low levels of residual C-peptide (0.09–0.60 ng/mL (0.030–0.200 pmol/mL)) in a random sample provide protection from severe hypoglycemia independent of HbA1c or insulin dose. 91

(A) Relationship of residual MMTT peak C-peptide (negative, <0.02 ng/mL; low, 0.05–0.60 ng/mL; intermediate, >0.60–1.20 ng/mL; and high, >1.20 ng/mL) and time spent in glucose range 70–180 mg/dL by continuous glucose monitoring. (B) Relationship of islet transplant MMTT peak C-peptide (low, <0.60 ng/mL; moderate, 0.60–<1.50 ng/mL; good, 1.5-to–<3.0 ng/mL; and excellent, ≥3.0 ng/mL) and time spent in the glucose range of 54–180 mg/dL by continuous glucose monitoring. Data are from Refs. 89 (A) and 108 (B).
Islet reserve and function with replacement in long-standing clinical disease
Long-standing (>10–15 years) symptomatic T1D is inextricably linked not only to decreasing prevalence of physiologically significant pancreatic islet cell reserve but also the development of progressive defects in glucose counterregulation to defend against the development of hypoglycemia. Initially, once insulin-deficient diabetes is established, the maintenance of an endogenous glucose production (EGP) response to defend against the development of low blood glucose appears most dependent on an intact epinephrine response rather than a relative increase in glucagon secretion. 89 However, it is well established that exposure to iatrogenic hypoglycemia associated with intensive insulin therapy reduces the glycemic threshold and magnitude of subsequent sympathoadrenal responses to hypoglycemia 92 , 93 such that with repeated hypoglycemia exposure, these may fail to reverse low blood glucose and contribute to a cycle whereby hypoglycemia begets further hypoglycemia with the development of impaired awareness and severe events. 94
Impairment of counterregulatory epinephrine and autonomic symptom responses to hypoglycemia contributes to impaired awareness of hypoglycemia, which combined with defective glucose counterregulation (impaired EGP response), leads to the development of hypoglycemia-associated autonomic failure (HAAF), also known as hypoglycemia unawareness, 95 which increases the risk of experiencing life-threatening severe hypoglycemia 20-fold. 96 Replacement of islet mass through allogeneic β cell transplantation in individuals with long-standing symptomatic T1D complicated by hypoglycemia unawareness restores physiologic islet cell responses to hypoglycemia that are associated with recovery of glucose counterregulation 97 and reversal of HAAF. 98 Thus, islet allotransplantation can provide additional insight into the relationship between functional islet reserve and glycemic control in T1D.
Islet allotransplantation is indicated for individuals with T1D and hypoglycemia unawareness experiencing severe hypoglycemia and/or excessive glycemic variability refractory to medical management with intensive insulin therapy. Islet allotransplantation involves the isolation of human islets from one or more deceased donor pancreata, which are transplanted by intraportal infusion during a percutaneous or minilaparotomy procedure for islet engraftment within the liver parenchyma. 99 , 100 Insulin independence is achieved when functional β cell mass is restored to >25–40% of normal based on measures of β cell secretory capacity derived from glucose potentiation of glucagon- or arginine-induced insulin secretion. 101 , 102 Lower estimates of functional β cell mass/secretory capacity following islet transplantation, while associated with ongoing insulin requirements, are related to proportionate improvement in glycemic control and variability relative to pretransplantation. Islet grafts with a functional β cell mass <5% of nondiabetic controls do not demonstrate any benefit on glycemic variability. 103 Continued islet graft function with reduction in severe hypoglycemia and glycemic variability is observed in 90% of recipients at 5 years; however, a decline in islet graft reserve capacity is often observed over time, with the majority returning to exogeneous insulin therapy by 5 years. 104 By contrast, although associated with significantly greater periprocedure morbidity, allogeneic whole pancreas transplantation more often results in insulin independence, and when transplanted simultaneously with the kidney from the same deceased donor is associated with a 5-year insulin independence rate >70%. 105 Assessment of β cell secretory capacity may require consideration for the systemic hyperinsulinemia that results from systemic venous drainage of the pancreas graft (reviewed by Rickels 106 ), although functional β cell mass/secretory capacity has been shown to be normal in recipients with portal venous drainage of the pancreas graft. 107 Restoration of a normal reserve capacity for insulin secretion likely explains the more durable insulin independence experienced by recipients of the whole pancreas when compared with isolated islet transplantation.
Incremental improvements in MMTT-stimulated C-peptide following islet allotransplantation are associated with a progressive reduction in glycemic variability and increasing time spent in the target glucose range by continuous glucose monitoring. 108 A continuous relationship was seen between stimulated C-peptide and all continuous glucose monitoring parameters, with a 0.100-pmol/mL increment in C-peptide associated with a 5% reduction in glucose standard deviation and a 2.5% reduction in interstitial glucose. Improved glycemic control (HbA1c) with a simultaneous reduction in exogenous insulin requirement was also observed across C-peptide groupings. Low levels (<0.6 ng/mL (<0.200 pmol/mL)) of MMTT-stimulated C-peptide following islet allotransplantation were associated with poor glycemic control and excessive glucose variability, with time in the range of 54–180 mg/dL (3–10 mmol/L) improving with moderate (0.6–1.5 ng/mL (0.200–0.500 pmol/mL)) and more significantly with good (1.5–3.0 ng/mL (0.500–1.000 pmol/mL)) levels ( Fig. 5B ). MMTT-stimulated C-peptide (>3.0 ng/mL (>1.000 pmol/mL)) was associated with 95% of the time within the 54–180 mg/dL glucose range and attainment of insulin independence. 108 Importantly, the restoration of only moderate levels of MMTT-stimulated C-peptide above the DCCT threshold of 0.6 ng/mL (0.200 pmol/mL) was associated in this high-risk cohort with absolute prevention of biochemical hypoglycemia (glucose <54 mg/dL). 108 Thus, the goal for interventions targeting preservation or restoration of β cell function in T1D should consider aiming for a stimulated C-peptide level of at least 0.6 ng/mL (0.200 pmol/mL) as suggested by recent consensus guidelines for defining outcomes of β cell replacement therapies. 109
Conclusions
Abnormalities in pancreatic islet β cell function and secretory capacity can be detected before the clinical presentation of T1D and progress over the years following diagnosis. It is now clear that residual islet β cells may persist for many decades with evidence in some cases of physiologic islet β cell function and that individuals with high residual C-peptide production may benefit from reduced glucose variability and improved overall glycemic control. Effective approaches to both preserve and restore pancreatic islet reserve before or at diabetes onset and sustain or replace the functional β cell mass in established diabetes are likely to lead to reduced diabetes-related complications with direct implications for quality of life. 110 Transplant studies have shown that even partial restoration of functional islet β cell mass can enable insulin independence, setting a target mass to attain through nontrans-plant approaches designed to maintain or regenerate endogenous pancreatic islet reserve.
Acknowledgments
The authors thank Henry T. Bahnson and Alyssa Ylescupidez of the Benaroya Research Institute for help with the creation of Figure 3 . M.R.R. is supported in part by Public Health Services Research Grants R01 DK091331 and UC4 DK112217 (Human Pancreas Analysis Program). This manuscript used data for Figure 2 acquired from the Human Pancreas Analysis Program (HPAP-RRID:SCR_016202) Database ( https://hpap.pmacs.upenn.edu ), a Human Islet Research Network (RRID:SCR_014393) consortium (UC4-DK-112217, U01-DK-123594, UC4-DK-112232, and U01-DK-123716). 111
Competing interests
M.R.R. has received consulting honoraria from Semma Therapeutics and Sernova Corp., and has received research support from Xeris Pharmaceuticals.
Weekly insulin injection as effective as daily injections in type 1 and type 2 diabetes, research suggests
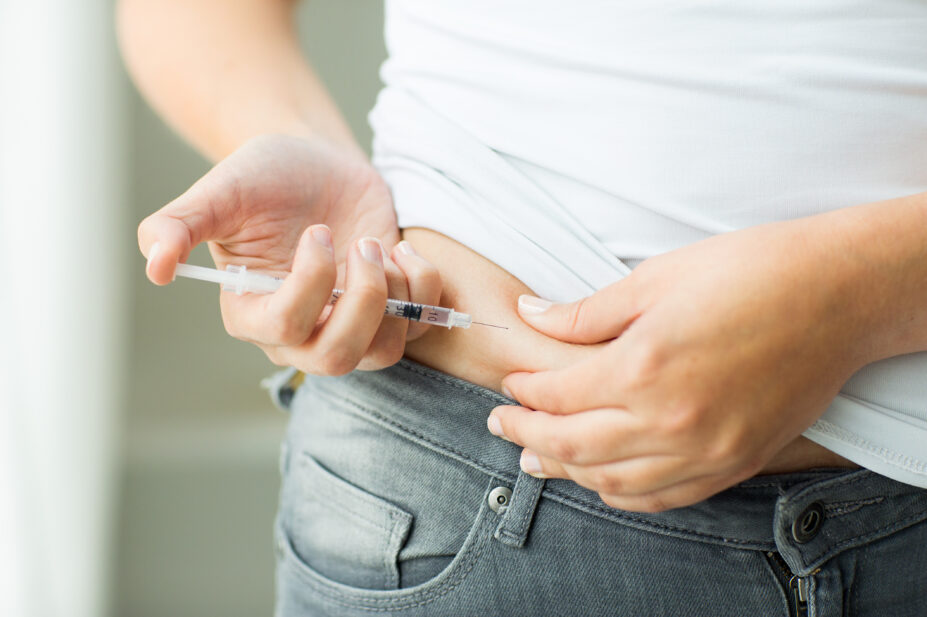
Shutterstock.com
A once-weekly injection of insulin is as effective as daily injections for blood sugar management in both type 1 and type 2 diabetes mellitus (T1DM/T2DM), studies have suggested.
In a phase 3, non-inferior study, published in The Lancet on 10 September 2024 , 692 adults with T1DM were randomly assigned to receive either once-weekly insulin efsitora (n=343) or once-daily insulin degludec (n=349), both in combination with insulin lispro.
The study aimed to assess the efficacy and safety of efsitora compared with degludec by measuring the change in glycated haemoglobin (HbA1c) and incidence of severe hypoglycaemia during the 52-week study period.
At week 26, researchers found that mean HbA1c decreased from 7.88% (62.66 mmol/mol) at baseline to 7.41% (57.5 mmol/mol) with efsitora, compared with 7.94% (63.3 mmol/mol) at baseline to 7.36% (56.9 mmol/mol) with degludec — a mean change of -0.51% with efsitora and -0.56% with degludec — confirming a non-inferiority margin of 0.4% for efsitora compared with degludec.
However, the incidence rate of moderate or severe hypoglycaemia with efsitora compared with degludec was 14.03 versus 11.59 events per patient-year of exposure — an increased risk of 21%, which was statistically significant.
The incidence of severe hypoglycaemia was also reported more frequently in the efsitora cohort at 10% (n=35/343), compared with degludec at 3% (n=11/349), during the 52-week period. Hypoglycaemia was reported more frequently in the first 12 weeks of the trial.
The authors noted that while efsitora showed non-inferior HbA 1c reduction compared with degludec, the greater incidence of severe hypoglycaemia in participants treated with efsitora may “suggest the need for additional evaluation of efsitora dose initiation and optimisation in people with type 1 diabetes”.
Another phase 3, non-inferior study, published on 10 September 2024 in the New England Journal of Medicine , included 928 participants with T2DM, who were randomly assigned to receive either efsitora (n=466) or degludec (n=462) over 52 weeks.
At week 52, researchers found that mean HbA1c decreased from 8.21% at baseline to 6.97% with efsitora (−1.26 percentage points) and from 8.24% to 7.05% with degludec (−1.17 percentage points), with an estimated treatment difference of −0.09 percentage points, demonstrating non-inferiority of the weekly injection.
The T2DM study also observed an incidence rate of moderate or severe hypoglycaemia with efsitora compared with degludec at 0.58 versus 0.45 events per participant-year of exposure; however, these findings were not statistically significant.
Commenting on the studies, Philip Newland-Jones, consultant pharmacist in diabetes and endocrinology at University Hospital Southampton NHS Foundation Trust, said: “Once-weekly insulin has the potential to support in those patients who have insulin resistance in T2DM and require similar background doses of insulin daily.
“There are possible groups that may benefit from once-weekly dosing, such as those requiring district nurses; however, there are also potential risks to take into consideration in this group,” he added.
“I believe that in line with data for insulin icodec once-weekly insulin, these data confirm that these insulins are unlikely to be used widely in T2DM because of the increased risk of hypoglcyaemic episodes compared with standard care.
“This is likely to be due to the differing daily requirements and flexibility in insulin required in the management of T1DM, further highlighting the benefits of hybrid closed-loop insulin systems, which can vary background insulin requirements,” explained Newland-Jones.
Similar to insulin efsitora, insulin icodec is a once-weekly injection, which is currently under review for regulatory approval for treatment of T2DM . However, it has also been associated with an increased risk of hypoglycaemia.
Guidance from the National Institute for Health and Care Excellence on icodec is expected to be published in May 2025.
Also commenting on the studies, Katie Bareford, senior clinical advisor at Diabetes UK, said: “Keeping blood sugars in target range with insulin therapy can be relentless and exhausting.
“A reduction in the number of insulin injections could lessen the burden of living with diabetes and better support people in their efforts to manage their diabetes.
“We welcome these findings and look forward to further research on once-weekly insulin injections to ensure they are safe and effective for everyone who could benefit,” she said.
Please leave a comment Cancel reply
You must be logged in to post a comment.
You may also be interested in
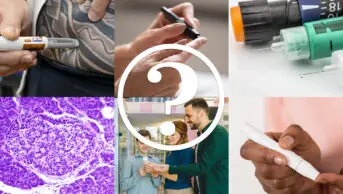
Test your knowledge of diabetes
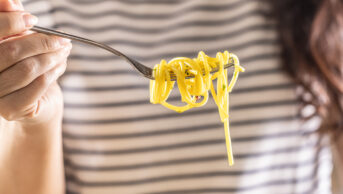
Almost one-third of people on low-calorie diet programme in remission from type 2 diabetes mellitus, study results show
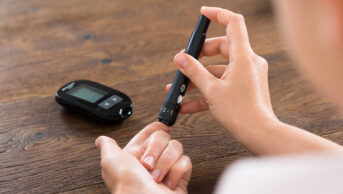
Number of patients at risk of type 2 diabetes increases by half a million in one year

IMAGES
VIDEO
COMMENTS
A suggested roadmap for future type 1 diabetes (T1D) research. Summary of priorities for T1D research as described in detail in the main text. ... Naji C, Teff KL.2005 β-Cell function following human islet transplantation for type 1 diabetes. Diabetes 54 100-106. ( 10.2337/diabetes.54.1.100) [Google Scholar] Rickels MR ...
We searched MEDLINE, Embase, and Scopus for research articles reporting long-term outcomes following allogeneic pancreatic islet transplantation published between Jan 1, 2000, and May 1, 2023, using the search terms "pancreatic islet transplant" AND "islet transplantation" AND "islet transplant" AND "donor Type 1 diabetes ...
A type 1 diabetes genetic risk score predicts progression of islet autoimmunity and development of type 1 diabetes in individuals at risk. Diabetes Care 41 , 1887-1894 (2018).
This concept has particular appeal for type 1 diabetes, a condition in which the loss of approximately 0.8 g of pancreatic β cells results in a lifelong need for insulin injections. Added to this, type 1 diabetes can be reversed by a pancreas or islet cell transplant, which provides proof of principle for cell-based therapy . Whole pancreas ...
This includes research to advance glucose management technologies, such as artificial pancreas devices that automate insulin delivery in response to blood glucose levels, as well as to develop cell-based approaches, such as islet transplantation, to replace the insulin-producing β (beta) cells that have been destroyed in type 1 diabetes. In ...
Type 1 diabetes results from the poorly understood process of islet autoimmunity, which ultimately leads to the loss of functional pancreatic beta cells. Mounting evidence supports the notion that the activation and evolution of islet autoimmunity in genetically susceptible people is contingent upon early life exposures affecting the islets, especially beta cells. Here, we review some of the ...
"We are hopeful that this first-of-its-kind research could be a gamechanger for the treatment of type 1 diabetes." As a result of these positive safety and efficacy data in Parts A and B, an independent data review committee has recommended moving to Part C of the trial, which allows for concurrent dosing of patients at the full target dose ...
The development of protocols for isolation of human islets and their improvement for treatment of type 1 diabetes by islet transplantation have enhanced access to isolated human islets for research 1.
Felton et al. conduct a systematic review to determine the utility of islet autoantibodies as biomarkers of type 1 diabetes heterogeneity. They find that islet autoantibodies are most likely to be useful for patient stratification prior to clinical diagnosis. ... Introduction. Type 1 diabetes ... and has research funding from a Diabetes UK ...
There is a growing understanding that the early phases of type 1 diabetes (T1D) are characterised by a deleterious dialogue between the pancreatic beta cells and the immune system. ... Islet cells in human type 1 diabetes: from recent advances to novel therapies - a symposium-based roadmap for future research J Endocrinol. 2023 Aug 31;259(1 ...
The presence of islet autoantibodies for a presymptomatic period of variable duration in first-degree relatives of individuals with type 1 diabetes has been known for more than 40 years (), with recommendations for islet autoantibody screening appearing soon after ().Decades of subsequent research and monitoring of individuals with islet autoantibody positivity has led to the paradigm shift ...
Pancreatic islets contain beta cells that produce the hormone insulin. In the type of islet transplantation used to treat type 1 diabetes, also called islet allo-transplantation, doctors take islets with healthy beta cells from the pancreas of a deceased organ donor. Doctors then inject the healthy islet cells taken from the donor into a vein ...
The rise of single-cell sequencing technologies has steered much of the recent SC-islet research toward thoroughly characterizing their transcriptional and chromatin landscape. Single ... Pancreatic islet transplantation in type 1 diabetes: 20-year experience from a single-centre cohort in Canada. Lancet Diabetes Endocrinol. 2022; 10:519-532.
Pancreatic islet cell transplantation successfully treated people with difficult cases of type 1 diabetes, although there were side effects. ... The research was supported by NIH's National Institute of Allergy and Infectious Diseases (NIAID), National Institute of Diabetes and Digestive and Kidney Diseases (NIDDK), and other NIH components. ...
Randomised controlled trials in which islets are transplanted into the portal vein of patients with type 1 diabetes with problematic glycaemic control ... Several areas for future research remain. ... De novo donor-specific HLA antibodies are associated with rapid loss of graft function following islet transplantation in type 1 diabetes. Am J ...
Affiliations. 1 Department of Diabetes and Cancer Discovery Science, Arthur Riggs Diabetes and Metabolism Research Institute, Beckman Research Institute, City of Hope, Duarte, CA, USA. Electronic address: [email protected]. 2 Department of Diabetes and Cancer Discovery Science, Arthur Riggs Diabetes and Metabolism Research Institute, Beckman ...
Keywords: islet autoantibody, type 1 diabetes, prediction, antibody assay. Type 1 diabetes (T1D) is an autoimmune disease characterized by hyperglycemia and metabolic disorder, which is caused by the destruction of insulin-producing pancreatic β-cells and absolute insulin deficiency [1]. Individuals with T1D depend on external insulin ...
Today, the U.S. Food and Drug Administration approved Lantidra, the first allogeneic (donor) pancreatic islet cellular therapy made from deceased donor pancreatic cells for the treatment of type 1 ...
"Although islet transplantation remains experimental, we are very encouraged by these findings, as we are by the rapid improvements in other treatments to help people with type 1 diabetes monitor and manage their blood glucose, including artificial pancreas technology." All 48 study participants received at least one islet transplant.
Like many autoimmune diseases, Type 1 diabetes stems from effector T cells gone rogue. The immune cells launch an attack on the insulin-producing beta islet cells in the pancreas, leaving patients ...
All health professionals involved in monitoring and care of individuals with type 1 diabetes have a responsibility to provide education. The guidance also emphasises "significant unmet needs for further research on early-stage type 1 diabetes to increase the rigour of future recommendations and inform clinical care".
Type 1 diabetes results from destruction of the insulin-producing β-cells in the pancreatic islets ().The majority of patients carry the HLA DRB1*03-DQB1*0201 or DRB1*04-DQB1*0302 susceptibility haplotype or both, but these are not sufficient for development of disease. For many years, viral infections have been suspected to play a role, but the specific etiologic agent(s) in human type 1 ...
Type 1 diabetes (T1D) is a disease of insulin deficiency characterized by autoimmune destruction of pancreatic islet β cells. Now, 100 years since the discovery of insulin in 1921-1922, the clinical course of T1D has been transformed. However, insulin therapy by injection or subcutaneous infusion informed by ongoing glucose monitoring ...
A once-weekly injection of insulin is as effective as daily injections for blood sugar management in both type 1 and type 2 diabetes mellitus (T1DM/T2DM), studies have suggested. In a phase 3, non-inferior study, published in The Lancet on 10 September 2024, 692 adults with T1DM were randomly assigned to receive either once-weekly insulin efsitora […]