
An official website of the United States government
The .gov means it's official. Federal government websites often end in .gov or .mil. Before sharing sensitive information, make sure you're on a federal government site.
The site is secure. The https:// ensures that you are connecting to the official website and that any information you provide is encrypted and transmitted securely.
- Publications
- Account settings
- Browse Titles
NCBI Bookshelf. A service of the National Library of Medicine, National Institutes of Health.
Institute of Medicine (US) Forum on Microbial Threats. Global Issues in Water, Sanitation, and Health: Workshop Summary. Washington (DC): National Academies Press (US); 2009.


Global Issues in Water, Sanitation, and Health: Workshop Summary.
- Hardcopy Version at National Academies Press
4 Addressing Risk for Waterborne Disease
Contributors to this chapter discuss a broad range of responses to the threat of waterborne disease, including drinking water disinfection, increasing access to water, improving sanitation, and investment in and implementation of public health interventions. Among these, the most seemingly straightforward approach—water treatment—is actually far from simple, as Philip Singer, of the University of North Carolina at Chapel Hill, demonstrates in the chapter’s first paper. Singer provides a quantitative overview of water quality and disinfection, emphasizing the use of chlorine as a disinfectant. He describes water quality factors (e.g., reduced inorganic material, dissolved organic carbon, and microbial contents) that influence chlorine’s effectiveness, and explains how sanitary engineers use the concept of “chlorine demand” to assess and address these factors in order to achieve water disinfection with chlorine. He also discusses parameters and limitations of various approaches to water treatment, including solar radiation, giving special attention to the significant barrier to disinfection posed by particulate matter and its removal by various filtration and flocculation methods.
In the developing world, the profound disease burden attributed to diarrhea makes it the most important target for waterborne disease prevention, according to workshop speaker Thomas Clasen of the London School of Hygiene and Tropical Medicine. Following a systematic review of interventions to improve water quality for preventing diarrheal disease ( Clasen et al., 2007a ), which compared interventions at the both the source (protected wells, bore holes, and distribution to public standpipes) and in the household (improved water storage, solar disinfection, filtration, and combined flocculation-disinfection), he and coauthors concluded that household-based interventions were nearly twice as effective as source-based measures. Clasen and coworkers subsequently conducted a cost-effectiveness analysis to determine the cost per disability-adjusted life year (DALY, a measure of disease burden) averted for a similar range of source and household interventions ( Clasen et al., 2007b ). The researchers found that upon reaching 50 percent of a country’s population, interventions involving household chlorination and solar disinfection paid for themselves and that all interventions were cost-effective.
The most prevalent method of home water treatment worldwide, boiling, was not included in these analyses. Although highly effective in reducing microbiological contamination, boiled water can be readily recontaminated; moreover, Clasen noted, boiling is relatively costly, is associated with risk for burn accidents, and results in indoor air pollution as well as carbon emissions ( Clasen, 2008 ). Because of boiling’s prominence, Clasen’s group has conducted assessments of its microbiological effectiveness and cost in several developing country settings in order to establish a benchmark against which other safe drinking water interventions can be compared. For example, in a recent study in semirural India, where more than 10 percent of households disinfect their drinking water by boiling, the researchers found that boiling, as practiced in these communities, significantly improves the microbiological quality of water (on a par with water filters), but does not fully remove the potential risk of waterborne pathogens ( Clasen et al., 2008 ). They also calculated that while the entry costs of boiling are the least of any water treatment option in this setting, the cost of continuing the practice annually is greater than the ongoing out-of-pocket cost of treating the same volume of water with sodium hypochlorite, or solar disinfection, and the five-year cost of boiling would also exceed most filtration options.
Efforts to increase the availability, uptake, and correct, consistent use of household water treatment and safe storage systems are spearheaded by the International Network to Promote Household Water and Safe Storage, a consortium of interested UN agencies, bilateral development agencies, international nongovernmental organizations (NGOs), research institutions, international professional associations, and private sector and industry associations ( Clasen, 2008 ; WHO, 2008 ). The Network now claims more than 100 members from government, UN agencies, international organizations, research institutions, NGOs, and the private sector and has accomplished much in terms of advocacy, communication, research, and implementation. However, despite these achievements, the mission of the Network to “achieve a significant reduction of waterborne disease, especially among children and the poor” is far from realization. Presently, only a tiny fraction of the millions of people who could benefit from household water treatment and safe storage (HWTS) interventions—far more than the one billion who use “unimproved” water sources, as previously noted—are being served, and those who need them most are the most difficult to reach.
In a recent report authored for the World Health Organization (WHO), Clasen (2008) examined efforts to scale up other important household-based interventions (e.g., oral rehydration salts, treated mosquito nets) for lessons of potential value to scaling up HWTS. He found several important recurring themes applicable to scaling up HWTS. These include the need to
- focus on the user’s attitudes and aspirations;
- take advantage of simple technologies (minimize behavior change);
- promote nonhealth benefits, such as cost savings, convenience, and aesthetic appeal;
- use schools, clinics, and women’s groups to gain access to more vulnerable population segments;
- take advantage of existing manufacturers and supply channels to extend coverage;
- provide performance-based financial incentives to drive distribution;
- align international support and cooperation to encourage large-scale donor funding;
- use free distribution to achieve rapid scale-up and improve equity;
- use targeted subsidies, where possible, to leverage donor funding; and
- encourage internationally-accepted standards to ensure product quality.
In his workshop presentation, Clasen noted that all introductions of novel health interventions to low-income populations face similar challenges—creating awareness, securing acceptance, ensuring access and affordability, establishing political commitment, addressing sustainability—but several additional barriers exist that must be overcome to scale up HWTS. These include
- the widely held belief that diarrhea is not a disease;
- skepticism about the effectiveness of water quality interventions;
- technology shortcomings with the available interventions;
- need for correct, consistent, sustained use (as compared with one-time interventions, such as vaccines);
- the existence of several transmission pathways for waterborne disease;
- suspicion on the part of the public health sector regarding the commercial agenda and lack of standards governing HWTS products;
- the orphan status of HWTS within governmental ministries; and
- the lack of focused international commitment and funding for diarrheal diseases.
“The goal of scaling up HWTS will not be achieved simply by putting more resources into existing programmes or transitioning current pilot projects to scale,” Clasen (2008) concludes.
The gap between where we are and where we need to be is to great given the urgency of the need. What is needed is a breakthrough. The largely public health orientation that has brought HWTS to its present point now need to enlist the help of another group of experts: consumer researchers, product designers, educators, social entrepreneurs, micro-financiers, business strategists and policy advocates. The private sector is an obvious partner; they not only possess much of this expertise but also the incentive and resources to develop the products, campaigns and delivery models for creating and meeting demand on a large scale. At the same time, market-driven, cost-recovery models are not likely to reach vast populations at the bottom of the economic pyramid where the disease burden associated with unsafe drinking water is heaviest . . . mass coverage among the most vulnerable populations may be impossible without free or heavily subsidized distribution. For this population segment, the public sector, UN organizations and NGOs who have special access to these population segments must engage donors to provide the necessary funding and then demonstrate their capacity to achieve both scale and uptake. Governments and international organizations can also help encourage responsible action by the private sector by implementing performance and safety standards and certification for HWTS products; reducing barriers to importation, production and distribution of proven products; and providing incentives for reaching marginalized populations. ( Clasen, 2008 )
Many of the ideas raised by Clasen regarding appropriately scaled water and sanitation infrastructure for developing countries are expanded upon by workshop speaker Joseph Hughes and coauthors, who offer an engineer’s perspective on water infrastructure in the developing world in the chapter’s second paper. Caravati et al. envision a new model for water and sanitation infrastructure that addresses global complexities, rather than a “one size fits all” approach based on developed-world systems. The authors describe several promising technologies that may help to address water and sanitation challenges in developing countries. First, however, they provide comprehensive background information on the dynamics of natural water movement, as well as the passage of water through the “engineered hydrologic cycle” of water and wastewater collection, treatment, and distribution.
Conventional, developed-world water and sanitation technologies “are often chemical-, energy- and operational-intensive, are based on heavy infrastructure systems (i.e., dams, pumps, distribution grids, etc.), and require considerable capital and maintenance, all of which hinder their use in much of the world,” the authors note. “If safe water and appropriate sanitation are to become accessible to those who are not currently served, new approaches and modern technologies must be employed. This will require a significant change in the way water and wastewater treatment systems are conceived and how they interact with other infrastructures systems (i.e., energy).” They outline a “new paradigm” for water and sanitation infrastructure and describe how progress under way in three vital areas—increased energy efficiency, availability of capital for business creation, and technology development—can advance this paradigm. Their contribution concludes with a review of research needed to fully develop a new, globally-appropriate model for water and sanitation infrastructure.
Given the global trend toward urbanization, particular attention must be paid to water and sanitation challenges for humans—tens of millions of them in megacities—living in close proximity to each other. The chapter’s third contribution, by workshop speaker Pete Kolsky of the World Bank and coauthors Kristof Bostoen and Caroline Hunt, focuses on the complex relationships that must be understood in order to recognize and address the threat of waterborne disease in urban settings, particularly in low-income communities. This essay originally appeared as a chapter in the book Scaling Urban Environmental Challenges: From Local to Global and Back ( Marcotullio and McGranahan, 2007 ).
Bostoen et al. begin by reviewing the effects of water supply, sanitation, and hygiene on health as viewed through two common models that clarify the complex interrelationships among these elements: classifications of water-related infections (see also Bradley in Chapter 1 ) and the F-diagram (depicted in Figure WO-13 ), a model of fecal-oral disease transmission. They then examine goals set by the international community for water and sanitation, along with obstacles that must be overcome in order to meet these goals, including the need to develop reliable measures of progress toward these goals. Following an examination of the significance of boundaries—“limits beyond which and individual or group feels no responsibility”—to urban water and sanitation issues, the authors conclude that institutional boundaries (which are central to many enviromental problems) must be identified and acknowledged. Improvements in water and sanitation services are significant only if they lead to change at the household level, they contend; therefore, household access to these services must be monitored and evaluated.
Ultimately, the threat of waterborne disease must be addressed through investment in safe water and sanitation interventions. Such investments are drastically underfunded, according to workshop speaker Vahid Alavian of the World Bank, who noted that the annual investment in water and sanitation needed to meet the MDGs exceeds $25 billion; only about half that sum is currently being spent. The World Bank is the largest global investor in water/sanitation investment, he added, but its portfolio of about $11 billion cannot begin to meet demand. His colleague Kolsky pointed out that the World Bank’s water and sanitation program at the Bank has received a grant of $20 million from the Bill and Melinda Gates Foundation to support sanitation scale-up and hygiene promotion projects, of which a significant fraction (15 to 20 percent) will be spent to evaluate the effectiveness of scaled-up interventions.
The chapter’s final essay, by speaker Sharon Hrynkow of the National Institute of Environmental Health Sciences (NIEHS) introduces a potential engine to drive the improvement of water quality and access in low-income settings: the phenomenon of social entrepreneurship. Using illustrative examples, she contrasts the social entrepreneur’s approach to solving these problems by focusing on delivering interventions or gathering information for policy purposes with that of medical researchers, who attempt to identify connections between toxins or microbes and illness, and then to reduce human exposure to disease agents.
“Increasing the dialogue between the medical research community and the social entrepreneur community would likely enhance operations on both sides,” Hrynkow concludes. In particular, she envisions an alternative to traditional medical grants, which rarely support policy development, that could support both medical research and social entrepreneurship and thereby encourage the transition of solutions for safe water and sanitation from basic science into practice.
- MEASURES OF WATER QUALITY IMPACTING DISINFECTION
Philip C. Singer, Ph.D. 1
University of North Carolina at Chapel Hill
This paper provides a discussion of important water quality factors impacting disinfection, with an emphasis on the use of chlorine as a disinfectant. It has been prepared to be somewhat tutorial in nature in an attempt to educate those unfamiliar with the complexities of water disinfection by chlorine. There are numerous textbooks with chapters on this subject ( Letterman, 1999 ; MWH, 2005 ).
Drinking Water Disinfectants
Several different types of disinfectants are used to treat drinking water:
- free chlorine (HOCl/OCl − )
- combined chlorine (i.e., monochloramine [NH 2 Cl])
- ozone (O 3 )
- chlorine dioxide (ClO 2 )
- ultraviolet (UV) irradiation
When chlorine is added to water, it hydrolyzes to form hypochlorous acid (HOCl) and the hypochlorite ion (OCl − ). Hence, free chlorine in water is a combination of HOCl and OCl − . Chlorine is the most widely used disinfectant for the purification of drinking water in the world. Ozone and chlorine dioxide are also used to disinfect drinking water in the United States, western Europe, and in some of the advanced Pacific Rim nations, but not in the developing world. UV irradiation—including simple solar irradiation methods employed in the developing world—is a growing technology to disinfect drinking water.
Disinfection Kinetics
Free chlorine is an effective disinfectant for inactivating waterborne bacteria, viruses, and a variety of protozoan cysts (e.g., Giardia ), but it is not effective against Cryptosporidium . Its effectiveness for inactivating microorganisms can be quantified under various conditions by a measure known as CT. 2 CT values are derived from the CT term in the Chick-Watson expression
in which N is the number concentration of microorganisms, k is a rate constant, C is the concentration of the disinfectant, and T is time. Integration of Equation (1) yields the log of inactivation as a function of the concentration of disinfectant multiplied by the contact time, expressed in units of milligram-minutes per liter.
The rate constant, k, depends on the specific disinfectant, the type of organism, and temperature. N o is the initial concentration of organisms. Requisite CT values to achieve various degrees of inactivation are temperature-dependent.
Table 4-1 shows CT values for the inactivation of Giardia and viruses by chlorine over a range of temperatures. In water at 5°C, at a concentration of 1 milligram per liter (mg/L) of chlorine, it will take 149 minutes to achieve 3-log inactivation of Giardia . For colder waters, more chlorine and/or longer contact times are needed to achieve the same degree of inactivation. Table 4-1 also shows that the CT values for virus inactivation are smaller than those for Giardia , reflecting the fact that viruses are easier to inactivate with chlorine than Giardia. At residual chlorine levels of 0.2 to 0.3 mg/L under the same conditions, 3-log inactivation of Giardia will require on the order of 12.5 hours (not shown).
CT Values (mg-min/L) for Microbial Inactivation by Free Chlorine residual) (pH 7.0, 1.0 mg/L Cl 2 .
Factors Affecting Disinfection with Chlorine
Several factors, in addition to temperature, influence the disinfectant potency of chlorine. The pH is an important consideration because it determines the form of chlorine present (HOCl or OCl − ). Hypochlorous acid is a more potent disinfectant than the hypochlorite ion; therefore, disinfection tends to be more effective with decreasing pH.
Chlorine Demand/Reducing Agents
Because chlorine is also a good oxidant, its stability in water is influenced by the presence of reduced inorganic and organic materials in the water, which exert a chlorine demand and chemically reduce the chlorine concentration. Additionally, the type and state of the microbial agents (i.e., whether the organisms occur as single cells or are associated with particles suspended in the water) affect the ability of chlorine to disinfect the water.
All of these factors determine the dose of chlorine that must be applied to a given water so that the target residual chlorine concentration (C) remaining at the end of a given contact time (T) can be achieved in order to meet the requisite CT value to ensure the desired degree of inactivation. The dose of chlorine applied, minus the chlorine residual, is known as the “chlorine demand” associated with a particular water supply. In a municipal water treatment facility, chlorine is usually applied to the raw water at the head of the treatment plant or after sedimentation or filtration, and the residual is measured at the point of entry to the distribution system. The difference between the dose and the residual is the chlorine demand and is due to consumption of chlorine by reduced organic and inorganic substances in the water. The higher the concentration of reduced organic or inorganic material, the greater the requisite chlorine dose needed to achieve a target residual and, hence, the greater the chlorine demand. In a village in which a woman collects water and carries it to her home where she adds chlorine to it, the chlorine demand reflects the amount of chlorine that must be added to the water in the container in order achieve the desired degree of inactivation in a specified time period, after which the water is presumed to be safe to drink.
To achieve the desired residual chlorine concentration to meet a target degree of inactivation as characterized by CT, one needs to calculate the dose of chlorine that must be added to any given water. To do this properly, one needs to know the degree to which reduced substances present in the water can lower the concentration of chlorine. This relationship is depicted in Figure 4-1 , which compares chlorine dose and residual free chlorine concentrations for several raw and partially treated waters (labeled here as MIEX ® effluents). The figure shows that, for the raw waters, 5–6 mg/L of chlorine must be applied in order to achieve a free chlorine residual of 1.0 mg/L. In this figure, the contact time is 24 hours. Hence, the chlorine demand of the raw water is 4–5 mg/L. For the treated waters, because a significant amount of dissolved organic material has been removed by treatment, the chlorine doses needed to achieve the same 1.0 mg/L free chlorine residual is 2–3 mg/L, reflecting a chlorine demand of 1–2 mg/L over 24 hours. Hence, in this case, treatment removed approximately 50 percent of the chlorine-demand associated with the dissolved organic material in the raw water.
Chlorine demand of several raw waters and partially treated waters (MIEX ® effluents). SOURCE: Reprinted from Boyer and Singer (2006) with permission from Elsevier.
Table 4-2 presents some examples of chlorine-demanding reactions with four inorganic reducing agents commonly found in raw water supplies: reduced (ferrous) iron (Fe(II)), (manganous) manganese (Mn(II)), sulfide (S(−II)), and ammonia (N(−III)). These balanced stoichiometric reactions illustrate the amount of chlorine that must be added to water to overcome the chlorine demand of these reducing agents. Iron, manganese, and sulfide typically derive from natural sources, whereas ammonia is often associated with municipal and agricultural discharges.
Chlorine Demand of Various Inorganic Reducing Agents.
Drinking water sources contaminated by sewage contain not only fecal bacteria and potentially pathogenic microorganisms but also organic material and ammonia, both of which exert substantial chlorine demands. As shown in Table 4-2 , the chlorine demand associated with ammonia is significant. Figure 4-2 depicts the progression of reactions that occur when increasing amounts of chlorine are added to water containing ammonia at a concentration of 0.5 mg/L as N. The first 2.5 mg/L of chlorine is converted to monochloramine; the next 2.5 mg/L of chlorine destroys the monochloramine. After this breakpoint is reached, free chlorine concentrations increase at essentially a 1:1 ratio as more chlorine is added. Thus, in order to get a free chlorine residual (the concentration of free chlorine beyond the breakpoint) necessary to meet the CT requirements for disinfection in water containing 0.5 mg/L of ammonia, at least 5 mg/L of chlorine must be added.
Breakpoint chlorination curve when chlorine is added to an ammonia-containing water. SOURCE: Reprinted from Water Chlorination/Chloramination Practices and Principles (M20) , with permission. (more...)
Natural organic material contains aromatic structures, unsaturated double bonds, and organic nitrogen, all of which react with chlorine. In addition to these oxidation reactions, chlorine participates in substitution and addition reactions to produce potentially carcinogenic halogenated byproducts. These include trihalomethanes, which are regulated in the United States by the Environmental Protection Agency and elsewhere in accordance with World Health Organization guidelines. On average, 1 to 1.5 mg/L of chlorine is consumed per mg/L of dissolved organic carbon (DOC) over the course of 24 hours, at pH 8 and 25°C.
Raw drinking waters generally contain a combination of chlorine-demanding impurities. A poor-quality surface water, for example, might contain 0.5 mg/L of ammonia and 6 mg/L of dissolved organic carbon, giving a total chlorine demand of 11-14 mg/L (5 mg/L to oxidize the ammonia in accordance with the breakpoint curve in Figure 4-2 and 6 to 9 mg/L for the 6 mg/L of dissolved organic carbon). For a better-quality surface water with 0.2 mg/L ammonia and 2 mg/L DOC, the chlorine demand would be 4-5 mg/L. For groundwater containing 1 mg/L iron, 0.5mg/L manganese, and 1 mg/L DOC, the chlorine demand would be on the order of 2.4 mg/L. Thus, different amounts of chlorine must be added in each case to achieve the same residual free chlorine levels needed for effective disinfection.
Measurement of Chlorine Residual
The most common method for measuring chlorine residual in treated water, the N,N -diethyl- p -phenylenediamine (DPD) colorimetric/spectrophotometric method, can distinguish between free and combined (monochloramine) chlorine species. However, because this method is subject to certain interferences, it must be performed and interpreted carefully, especially at low free chlorine concentrations and in waters containing dissolved organic nitrogen. Organic chloramines that are formed when free chlorine is added to water containing dissolved organic nitrogen, a component of the breakdown of proteinaceous material, also tend to react like free chlorine in the DPD colorimetric analysis. Because these organic chloramines do not have the disinfecting power of free chlorine, their presence gives an artificially high apparent free chlorine residual and a false sense of disinfection effectiveness. Additionally, while the minimum “detectable residual” with the DPD test is 0.2 mg/L chlorine, there are many instances where such detectable residuals have been measured by the DPD test but these waters have also been found to contain coliform bacteria that should not survive in the presence of chlorine at that concentration. Thus, measurements of free chlorine residual are subject to some uncertainty and must be interpreted carefully depending on water quality conditions.
Turbidity and Particle Content
The effectiveness of disinfection is impacted by the presence of particulate material in the water. Particles tend to protect the microorganisms from exposure to the disinfectant, especially if the microorganisms are present in an aggregated state. In the latter case, the organisms at the surface of the particle are exposed to the disinfectant but the organisms inside the aggregate are protected from exposure.
The turbidity of water is used as a surrogate for particle content. In the United States, water turbidity is monitored continually using a simple, relatively inexpensive device called a turbidimeter or nephelometer, which measures the light-scattering properties of particles at 90° to the incident light (see Figure 4-3 ). The intensity of scattered light is a function of the number, size, and shape of the particles present in the water (as well as of the wavelength of incident light, geometry and detection characteristics of the instrument, and its method of standardization and calibration). Water turbidity is therefore a collective reflection of a property of the particles (their light scattering characteristics) rather than a specific measure of particle size, number, or morphology.
Schematic of a nephelometer used to measure turbidity. SOURCE: Reprinted from http://www.eoc.csiro.au/instrument/html/marine/marine_images/hach_diagram.gif (accessed April 16, 2009) with permission from Hach Company.
In general, small particles scatter light more than larger particles, with the greatest degree of scattering resulting from particles that are about 0.5 microns (μm) in diameter, which is equivalent to the wavelength of the incident light. Viruses, which are much smaller in size (on the order of 0.03 μm), do not scatter light, whereas bacteria (0.5 to 1 μm in size), Cryptosporidium oocysts (3 to 5 μm), and Giardia cysts (10 to13 μm) do scatter light. Hence, the absence of a measurable turbidity does not guarantee that the water is free of harmful microorganisms.
A better measure of particle content can be achieved with particle size analyzers. A variety of particle size analyzers are commercially available for characterizing particles in water. Particle counters can measure particle size and concentration, providing information about size distribution (i.e., the number concentration of particles of various sizes) in the water. Optical methods (see Figure 4-4 ) compare light blockage by the different particles as a known volume of water is drawn through an orifice. The degree of blockage is proportional to the cross-sectional area of the particle. As particles of different size are drawn through the sensing zone of the instrument, the extent of blockage of the incident light is sorted into different channels according to the amount of light blocked, giving rise to a particle size distribution based on the diameter of an equivalent sphere with the same cross-sectional area. Resistivity-based methods, which measure the volume displacement of water by particles of different sizes in a salt solution, provide information on the size distribution of particles according to their volume-equivalent particle diameter.
Schematic of an optical particle counter. SOURCE: http://www.oilanalysis.com/backup/200207/PartCount-Fig1.jpg (accessed April 16, 2009) © Noria Corporation.
Image analyzers are recent additions to particle characterization techniques in water quality analysis. With these instruments, water samples can be continuously examined under a microscope, photographed, and the images stored in a computer file for subsequent review and analysis. These instruments permit identification of particle type and morphology. As previously noted, microorganisms in a sample may be present as single cells or as aggregates, and it is important to determine their degree of aggregation in order to assess their susceptibility to be inactivated by chlorine or any other disinfectant.
Particle Removal
Because microorganisms are often found in an aggregated state, and because particle-associated microorganisms are difficult to inactivate, the first line of defense against microorganisms of potential public health concern is filtration. Filtration removes particles, both particles that are present in an aggregate state and free, single-cell organisms which are themselves particulate in nature. Dis- infection with chemicals, such as chlorine, and physical inactivation methods, such as UV light, cannot be relied upon to safely disinfect water that has not undergone filtration. Effective filtration can occur via natural means (e.g., filtration that occurs by flow through porous media in a groundwater aquifer), engineered filtration (e.g., granular media filtration in a water treatment plant), or household filtration (e.g., filtration in a biosand or ceramic pot filter, both of which are being widely promoted for use in rural villages in developing countries). Once the raw water is relatively free of particulate material, chemical disinfectants or UV light can provide effective disinfection, provided the dose and contact time are sufficient.
- CIVIL INFRASTRUCTURE FOR WATER, SANITATION, AND IMPROVED HEALTH: EXISTING TECHNOLOGY, BARRIERS, AND NEED FOR INNOVATION
Kevin C. Caravati
Georgia Tech Research Institute
Zakiya A. Seymour 3
Georgia Institute of Technology
Joseph B. Hughes, Ph.D., P.E., BCEE 4
Introduction
Civil infrastructure can be broadly described as the systems, services, and facilities needed for a functioning community or society. Examples of easily recognized civil infrastructure include dams, bridges, buildings, roads, transmission and distribution lines, and communication technologies. These and other infrastructure systems, collectively, are central to the improvement of health, quality of life, and prosperity of communities. Among the most basic of all civil infrastructure systems are those that store, convey, treat, and provide potable water, as well as collect, treat, and safely discharge wastewater. Together, these systems have a dramatic impact on human health and the health of the environment in which people live.
Engineers and scientists have studied methodologies to purify water contaminated with biological, chemical, and physical contaminants for centuries, and well-accepted techniques for water and wastewater treatment have been employed in the United States and other parts of the developed world for many decades. Yet access to water and sanitation remains one of the largest challenges for societies around the globe. Of the world’s 6.5 billion-plus inhabitants, an estimated 1.2 billion people lack access to safe drinking water and 2.6 billion, or 42 percent of the world’s population, lack access to basic sanitation ( World Water Assessment Programme, 2006 ). Concerns of water access and improved sanitation are compounded by threats associated with climate change and a projected growth in population to 8 billion people by the year 2030. According to the Organisation for Economic Co-operation and Development (OECD), the number of persons in water-stressed countries is expected to increase to nearly four billion ( OECD, 2008 ). By 2025, more than half the nations in the world will face freshwater stress or shortages, and by 2050 up to 75 percent of an estimated 9.1 billion people could face freshwater scarcity ( Hightower and Pierce, 2008 ).
The future health, prosperity, and security of the human race will be strongly influenced by our ability to access clean water. Growing populations, rapid urbanization, expanding industrialization, changes in climate, growth in irrigated agriculture, and the globalization of corporations further contribute to our global water challenge. Infrastructure models deployed in the developed world over the past century are often ill suited to provide sustainable, scalable solutions. A new model is needed that addresses the complexities around global water and sanitation infrastructure.
Presented herein is an analysis of impediments to the solution of the world’s water and sanitation needs through existing technology. First, a background section is prepared to provide brief reviews of (1) the cycle of water in nature and (2) the cycle of water through engineered systems. Second, an examination of well-established water and wastewater infrastructure models detail a range of factors that exist as barriers to the translation of models globally. Finally, a discussion of the potential for technological innovations to provide advances in improving water and sanitation challenges in developing countries is presented.
Water is a widely occurring compound on Earth that is found in liquid, gaseous, and solid forms. Oceans cover approximately three-quarters of the Earth’s surface and contain over 97 percent of the world’s water supply. Oceanic water contains high salt concentrations and is unfit for human consumption without extensive treatment. Less than 3 percent of the Earth’s water is “fresh water,” and nearly 70 percent is in glaciers and icecaps, providing a mere 0.3 percent accessible for human consumption in lakes, reservoirs, rivers, and aquifers.
To understand water as a resource for human consumption, it is important to understand the dynamics of natural water movement among its three phases and the reservoirs for water that provide storage. Correspondingly, it is necessary to understand the flow, storage, and treatment of water through engineered systems used for the collection, treatment, and distribution of potable water and wastewater. For the purposes of clarity and consistency, the process of water movement and storage on Earth without human intervention is referred to as the “natural hydrologic cycle.” Water movement through infrastructure systems for the delivery of clean drinking water to the public is referred to as the “engineered hydrologic cycle.”
Natural Hydrologic Cycle
The largest reservoir for water is in the oceans. Evaporation 5 results in the transfer of water to the atmosphere. Condensation of gaseous water to the liquid state occurs within the atmosphere forming clouds and precipitation. A diagram of the natural hydrologic cycle is presented in Figure 4-5 . Roughly three-quarters of global precipitation results in water returning to the oceans. The fraction that falls on land initiates the terrestrial component of the water cycle. On land, water will be present as a solid (ice and snow) and as a liquid. The inflow, or precipitation that falls on land, is the predominant source of water required for human consumption, agriculture and food production, industrial waste disposal processes, heat dissipation in energy production, and for support of natural and seminatural ecosystems ( World Water Assessment Programme, 2003 ). Outflow of the natural water cycle includes drainage to the oceans via rivers, lakes, and wetlands; evapotranspiration of water to the atmosphere from soils by plants; and evaporation from fresh water reservoirs. Water that collects into flowing bodies of water is categorized as surface water (streamflow). Water that seeps through soils into underlying rock layers is contained as groundwater. Surface water systems are more rapidly recharged than groundwater. In fact, the flow of groundwater systems is very slow and the volume of water they can produce is often finite.
The hydrologic cycle. SOURCE: USGS (2008).
Engineered Hydrologic Cycle
Engineered water systems are processes that convey, store, and alter water quality. Many of these processes are designed to enhance what characteristically occurs in the natural environment (i.e., the breakdown of organic contaminants in wastewater by bacterial communities). A vast network interlinking water, sanitation, and energy infrastructure systems has been built to ensure clean drinking water and mitigate the impacts of waste on human and environmental health. The development and reliability of this network, collectively referred to as the “engineered hydrologic cycle,” are critical factors in the growth and health of populations. Major components to describe the details of this network consist of (1) water supply creation and protection, (2) water and wastewater treatment, and (3) water quality.
Creation and Protection of Water Supply
An estimated 48 percent of the freshwater supply in the United States is used for energy production. The remaining percentage is classified into irrigation (34 percent), public use (11 percent), industrial use (5 percent), and mining, livestock, and aquaculture (less than 2 percent; USGS, 2005 ). While the focus of this discussion is public use, the largest supply issues are driven by the energy and agriculture sectors.
Due to the scarcity of freshwater throughout the world, careful consideration must be given to create and protect water supplies. Over the past 200 years, human activities have developed to such an extent that only a few natural water bodies remain ( World Water Assessment Programme, 2003 ). Water management over the past century has focused on large-scale diversions of water out of natural systems; more than 60 percent of the world’s rivers have undergone major hydrological alterations ( Revenga et al., 1998 ). Throughout much of the developed world, large reservoirs with intake structures, dams, and distribution facilities provide clean water to millions over vast areas. Well fields that tap highly productive aquifers can do the same. Additional infrastructure changes also include extensive channelization of river systems, massive pumping of aquifers, and long-distance water conveyance systems. These engineered systems, representing the conventional approach for creating water supplies, have significantly altered lifestyles and the environment by ensuring the sustainability of water resources ( Gleick, 2006 ).
In conjunction with creating water supplies, the development of resource protection programs ensures water quality. Resource protection involves several stakeholders, including regulatory agents, governments, commercial users, and residential consumers. It comprises a variety of watershed protection practices, including restricting land use in sensitive areas, managing solid wastes, and preventing saltwater intrusion. Collectively, through the application of these methods and the cooperative assistance of involved stakeholders, water supplies are safeguarded and public health is protected. The New York City Watershed Partnership project, described in Box 4-1 , is an example of a successful large-scale water supply protection and infrastructure project. This partnership provides unfiltered drinking water to nine million people while preserving the economic viability and social character of the communities located in the upstate watershed ( EPA, 2006 ).
Unfiltered Drinking Water for Millions: The New York City Watershed Partnership. New York City’s water managers face two challenges: protecting the public health of both the city’s (more...)
Water and Wastewater Treatment
To ensure clean water supplies in the developed world, engineers have created treatment systems to provide safe drinking water from both surface water and groundwater sources, and to return the resulting wastewater to surface waters (on rare occasions water is returned to aquifers). These systems, which include drinking water and wastewater treatment facilities, conveyance systems, above-ground storage facilities, and residuals management sites, are necessary for providing water for public use as well as for disposing of wastewater and wastes in a safe and efficient manner. A graphic depiction of these water and wastewater treatment systems is shown in Figure 4-6 .
Detailed diagram of conventional water and wastewater treatment systems. SOURCE: Reprinted from ABB (2007) with permission, www.abb.com/water.
Initially, surface water and groundwater are directed to a drinking water treatment plant. The water is then treated to remove particulate matter (i.e., solids) and dissolved contaminants and is disinfected. Additional treatment for taste, appearance, hardness, and odor occurs before it is stored and/or piped to a distribution system. This distribution system delivers drinking water for consumption. After the public use, wastewater, through a collection system, is sent to a wastewater treatment plant for solids removal and biological and chemical treatment. Afterward, treated wastewater is discharged to a river, lake, reservoir, ocean, or aquifer system.
Water and wastewater treatment methodologies involve physical, biological, and chemical processes; these treatments are usually completed in series, and are, subsequently, also known as primary, secondary, and tertiary treatments. In potable water treatment, physical (i.e., filtration) and chemical (i.e., chlorination) are most common. In wastewater treatment, biological treatment is used to remove organic matter (i.e., biochemical oxygen demand or BOD) and treat residuals (i.e., anaerobic digestion). Physical treatment (i.e., gravity separations) and chemical treatment (i.e., chlorination) are also essential in wastewater treatment facilities. If the wastewater has been created in an industrial facility, additional treatment(s) may be required prior to its discharge. Listed in Table 4-3 , as modified from Tchobanoglous et al. (2003) and in Viessman and Hammer (1985) , are various methodologies that treat water and wastewater.
Conventional Water and Wastewater Treatment Methodologies.
Treating water before and after public use is essential; often, surface waters that receive wastewater discharges are typically a drinking water source for communities downstream. Practices implemented in one part of a watershed have the capacity to impact its potential use as a water source. Consequently, the conventional engineered solution for drinking water and wastewater treatment, as seen in the developed world, imparts its own engineered hydrologic cycle in concert with the natural water cycle, providing integrated resource protection to reduce vulnerability and maintain the quality of water sources.
Water Quality
The quality of water found in rivers, lakes, reservoirs, and groundwater depends on several linked factors. These factors include geology, climate topography, biological processes, land use, and water residence time. Nonetheless, the degree of sanitation practiced is the most critical determinant of contamination of drinking water with pathogens.
Programs dedicated to water and sanitation typically spend 95 percent of their resources on water ( Black, 2008 ), yet the global return on investments in low-cost sanitation provision may be in the range of $9 for each $1 spent ( Hutton et al., 2006 ). Human and agricultural wastes pose a remarkably high risk to waterborne disease. The principal bacteria that cause intestinal disease include several genera of Salmonella, Shigella, Vibrio cholerae, Leptospira , and Yersinia enterocolitica . An average bowel movement weighs 250 grams and an average human produces 77 pounds of excrement per year ( George, 2008 ); one gram of feces can contain 10 million viruses, 1 million bacteria, 1,000 parasite cysts, and 100 worm eggs ( George, 2008 ), carrying the potential to threaten any water source. In nature, there is no pure water, but water that has been tainted due to poor sanitation is particularly poor quality.
The measurements of certain physical, chemical, and biological water quality parameters assist in determining the suitability of water for various purposes, the effectiveness of water and wastewater systems, and its potential impacts on public health. Water quality regulations in the United States and other developed countries require that water be properly managed to improve human health and minimize environmental degradation. In the United States, the number of water quality parameters evaluated on a routine basis at water treatment and wastewater treatment plants is considerable. A short list of examples includes pH, dissolved oxygen, hardness, turbidity, BOD, nitrate, ammonia, color, fecal coliform bacteria, heavy metals, and toxic organic pollutants, among others. Table 4-4 , as provided by the American Water Works Association ( AWWA, 1999 ), summarizes the source and significance of certain water quality parameters.
Water Quality Parameters.
Established Water/Wastewater Infrastructure Models
The development of the engineered hydrologic cycle is the basis for establishing water/wastewater infrastructure models. For discussion purposes, currently established water/wastewater infrastructure models common in the United States are referred to as the “conventional infrastructure model.” Where traditional infrastructure models are not used, the term “household water treatment and storage model” is employed. Components of each model are discussed in the following section along with the barriers for increased implementation of both.
Conventional Water/Wastewater Infrastructure Model
The overall success of conventional water infrastructure in the developed world is attributed to the complementary advancements in engineered, economic, and societal systems since the mid-1800s. Conventional water and sanitation technologies, as seen in most developed regions, were mature decades ago—although through research engineers have continued to improve the performance of treatment systems. These technologies are often chemical-, energy-, and operational-intensive, are based on heavy infrastructure systems (i.e., dams, pumps, distribution grids, etc.), and require considerable capital and maintenance, all of which hinder their use in much of the world ( Shannon et al., 2008 ). The success of the U.S. water infrastructure improvements relies on several interrelated and critical elements. Examples are provided below:
- Energy Use . Energy consumption represents the largest operational cost in water and wastewater treatment. It is essential for source collection and conveyance systems, distribution systems, and treatment. It is also needed for pumps (within wells, at surface water intakes, and for distributing water), aerators, chemical feed systems, and biological treatment systems.
- Chemical Use . Water and wastewater treatment require large quantities of chemicals to treat water efficiently and to remove elements that may inhibit water quality. Chemicals are also used for corrosion control and disinfection within the distribution systems. Chemical use can include ammonia, chlorine compounds, antiscalants, ozone, permanganate, alum, ferric salts, sodium hydroxide, hydrochloric acid, and ion exchange resins.
- Subsidies. Subsidies are often required to ensure widespread acceptance and adoption of water supply and wastewater treatment to the public. Additionally, certain subsidies, such as those provided to the agricultural industry within the United States, can deeply impact the availability of water. Regulatory agencies and governments must consider the interests of the public.
- Regulatory Frameworks. Regulatory frameworks are needed to establish ownership rights, to set standards for drinking water and industrial waste-water treatment, to develop water permitting requirements, and to determine resource allocations (power generation, recreation, agriculture).
- Available Capital . Determining the upfront capital available for the design and construction costs of a water infrastructure system is essential. Furthermore, careful consideration must be given to the long-term operation and maintenance needs of the facilities. In most developed countries, these expenditures are typically financed by the public sector.
- Property Ownership. Obtaining the appropriate property rights-of-way is necessary to protect the components of the water infrastructure system, including the watersheds, reservoirs, piping, and distribution sites. Additionally, determining property ownership will provide useful knowledge for the system users and the ability to establish a payment system for water usage.
- Social Acceptance. While regulatory frameworks establish the legality frameworks, the public must also accept components of a water infrastructure system. There are several decisions made when developing a water infrastructure system, such as determining financing options, permitting restrictions, and treatment and sludge disposal methods. These alternatives highlight the complexity of decision matrices and stress the need for collaboration with the public.
Barriers for Expanded Application of Conventional Infrastructure Models
The components essential for building conventional water infrastructure systems often do not exist in developing countries. Reliable, consistent supplies of energy are required, and chemicals for treatment may not be obtainable. Governments in developing nations often lack the authority and resources to implement large-scale programs, or they may be plagued by corruption or governance issues. Organizations may be able to obtain the initial funding but may not have sufficient revenue collection systems needed for operational costs. Additionally, concerns exist about the development of water resources through major dam-building programs. This lack of resources, revenue, and regulation impedes the successful development of the conventional water infrastructure model in developing countries. Specific examples of barriers that effectively prohibit the reliable and effective use of conventional water and wastewater infrastructure systems are illustrated below.
Water supply stress Worldwide water supplies and the quality of freshwater are being impacted by climate change, demographic shifts, and population growth, creating regions experiencing water stress. The traditional solution to water stress has been to enhance the water supply through conveyance from increasingly distant sources. Often, these systems include well-field and water distribution networks, household connections for wastewater conveyance and treatment, and the installation of dams for combined hydroelectric power generation and water supply reservoirs. Yet, this approach is frequently found unreliable in developing countries due to environmental, social, and economic reasons ( Gleick, 2000 ). Traditional water management methods to divert water out of natural systems, if built, have not been maintained effectively; thus, much of the water supply is restricted to nearby surface water or shallow groundwater, making it more difficult to find and retain water supplies for croplands and urban centers. Both surface and groundwater supplies can become contaminated from human, industrial, and animal waste; depending on water demands, groundwater water tables can fall below acceptable recharge levels.
Concentrated population growth places a particular stress on water supplies. Over the next century, an additional three billion people will live in urban areas ( Zimmerman et al., 2008 ), further focusing water demand and waste production. Outside of urban areas, the loss of forests and vegetation to support urbanization causes increased sedimentation, loss of wetlands, and eutrophication ( Zimmerman et al., 2008 ). By the year 2025, water withdrawals are expected to increase from current levels by 50 percent in developing countries and 18 percent in developed countries ( Zimmerman et al., 2008 ). Demands from the agricultural, industrial, and energy sectors will compete with the needs of coastal developments; economic development will likely take precedence over resource protection. Lacking strong governance systems, agencies are subject to corruption and an inability to create or enforce environmental protection practices to ensure the sustainability of water resources.
Energy The lack of reliable energy is problematic for establishing conventional solutions in developing regions. Energy requirements for water and wastewater treatment are staggering. Without reliable energy systems, conventional treatment processes simply fail. Equally important to having energy to treat water will be the impact of energy systems on water stress. Growth in future energy production is projected to be highest in water poor regions. Furthermore, the regions with the highest increased projected energy demand will include coastal North and South America, the Middle East, India, and China. Increasing energy production will require a reevaluation of water resources and reduce freshwater availability.
Poor sanitation The effect of poor or nonexistent sanitation on water resources can overwhelm the ability of conventional water treatment systems to provide safe water. Without regulations, surface and groundwater systems are subject to contamination with fecal matter, rendering them unfit for use as a drinking water source without advanced treatment systems. For millions of people, water is simply too precious to be used for disposal of waste, therefore the waste will not enter or be conveyed through a sewer system even if one existed. Conventional wastewater treatment requires that waste be conveyed by water. In many locations that simply will not occur and the engineered water cycle is “short circuited.”
Economics and regulatory needs The price one actually pays for water is but a small fraction of what it truly costs to extract it, deliver it to users, and treat it after its use ( Revenga, 2009 ). Subsidies that hide the true costs of water or subsidies for programs that can pay for themselves have proven to be ineffective. Furthermore, governments are less willing to subsidize large dam or water infrastructure projects and are shifting more responsibility to regional and local governments ( Gleick, 2000 ).
Privatization of water services (and energy services) has been a “widespread failure” according to a recent United Nations Development Program study ( Bayliss and McKinley, 2007 ). Private investors have shied away from investing in public utilities, and the private enterprises have focused primarily on cost recovery and not the provision of services at equitable prices to the poor. Current efforts tend to focus on building the capacity of the public sector, but funding sources for such projects are scarce. Energy subsidies in India encourage ground-water users to pump more than they actually need, leading to massive declines in local and regional water tables ( Gleick, 2000 ). Agricultural subsidies in Asia encourage the consumption of inflows to the Aral Sea, resulting in shrinkage of the Sea and loss of species and livelihoods ( Gleick, 2000 ).
Policies and pricing decisions that do not effectively price water lead to misuse. The poor pay more for water that is delivered from private sources compared to water provided by a municipality ( World Water Assessment Programme, 2006 ). The World Health Organization defines reasonable access as the availability of at least 20 liters per capita per day from a source within one kilometer of the user’s dwelling ( WHO, 2008 ).
Regulatory frameworks are particularly important for sanitation services, but sanitation is typically underfunded. Waste disposal services are often nonexistent in poor rural areas or crowded urban centers or are unaffordable. Subsidies often are needed to promote access to utility services ( Bayliss and McKinley, 2007 ), and some utilities have introduced “lifeline tariffs” in which minimal levels of utility services are provided free or at low cost. Oftentimes, utility connections to poor neighborhoods are subsidized; water delivery from these connections needs to be safe and reliable; otherwise public trust in the local authorities is lost.
Issues of property ownership plague megaslums and rural areas, which complicates the delivery of water and energy services. The ability of dwellers or residents to pay for services and the ability to collect connection fees and monthly user fees can be barriers to improvements.
The adoption of a traditional water infrastructure system by many institutions has reached a plateau as environmental and social concerns have increased. Population growth and changing demographics have limited unbridled expansion opportunities that existed in the nineteenth and twentieth centuries. Financing of major projects has declined due to rising material costs, legal opposition from stakeholders, and now a tightened credit market.
Household Water Treatment and Storage Water Treatment Model
The most common household water treatment and safe storage systems include chlorination, filtration (biosand and ceramic), solar disinfection, combined filtration/chlorination, and combined flocculation/chlorination. Lantagne et al. (2006) provide a thorough review of these systems with a summary of performance criteria for each option. These systems focus on point-of-use or point-of-entry drinking water treatment and storage. Chlorination and flocculation/chlorination methods are scalable at the village and national level; scalability issues exist for biosand, ceramic, solar disinfection, and filtration/chlorination options. The adoption of household water treatment and storage systems has increased in developing nations in recent years. These systems are small-scale applications of certain basic processes in the engineered hydrologic cycle discussed previously, but the treatment is not as comprehensive as is typically done in conventional systems.
This model has been most successful when local organizations have the capacity to provide materials and replacement parts, can provide technical assistance, and can facilitate behavior change communications ( Lantagne et al., 2006 ). Significant challenges for these systems include evaluating the health impacts of these interventions in “real-world” settings, sustainability of the projects, and scalability in terms of reaching people without access to improved water sources ( Lantagne et al., 2006 ).
Barriers for the Household Water Treatment System Model
Community education is often necessary to ensure adoption of the intervention and to ensure community acceptance. These efforts are labor- and time-intensive, and success is measured in small steps. Cultural traditions can impact the adoption rates of practices that are generally accepted in the developed world. For example, in Western Cameroon, it is considered “taboo” by some to use household chlorination (locally known as “poison”) in drinking water (personal communication with P. Njodzeka of the Life and Water Development Group, Cameroon, December 30, 2008).
In rural farming villages, household water treatment systems are simply unaffordable to many where household income can be $0.25 per day or less, and residents collect water from springs or often drink from contaminated streams.
The adaptability rate of a household intervention is an area for further research. While knowledge of the intervention may be widespread, actual adoption rates may lag due to economic factors, a perceived lack of personal benefit from the intervention, and cultural or political issues.
A Paradigm Shift for Water and Sanitation Infrastructure
Variability exists in the designs and operation of conventional water infrastructure systems, but these generally follow a “prescriptive approach” based on Victorian age (or older) methodologies. Even if the capital and human will existed to create conventional systems for all people, it would take decades to build and it is uncertain that these systems are well suited to meet the demands of population growth and urbanization. If safe water and appropriate sanitation are to become accessible to those who are not currently served, new approaches and modern technologies must be employed. This will require a significant change in the way water and wastewater treatment systems are conceived and how they interact with other infrastructure systems (i.e., energy). Proposed features of a paradigm shift to address the complex challenges around global water and sanitation are shown in Table 4-5 .
Paradigm Shifts Addressing Water and Sanitation Infrastructure.
The Innovation Challenge
The benefits of conventional water infrastructure systems are undeniable. Human health has been improved as has life expectancy. Water supplies in most developed nations are relatively clean and reliable. Many of the water-related diseases rampant in Europe and North America in the late 1800s are no longer a concern in those regions ( Gleick, 2000 ). However, the implementation of these systems carried a tremendous economic cost, and they require a continuous investment in operations and maintenance. In addition, they have greatly disturbed many ecosystems, displaced populations, and created new health concerns such as the formation of trihalomethanes, a disinfection byproduct linked to cancer.
Research activities in the United States, and other parts of the developed world, have focused on advancing the water and sanitation approaches within the conventional water and wastewater treatment paradigm. By comparison, research and technology for the development of nonconventional water and sanitation has been very limited, and market penetration of many of the proposed solutions has been inadequate and difficult to sustain. In order to make rapid progress in solving the world’s water challenge, it is essential that research be directed at approaches that can be developed within the “new paradigm” presented earlier. In addition, research must be focused on water efficiency in the major water usage areas of energy and agriculture. Whether interest is directed to new treatment systems or to increased efficiency, there is reason to believe that new solutions are possible and with focused and sustained efforts, significant improvements in water access and sanitation can be realized. In this section, three specific areas where innovation yields interest today are presented. First, are changes in the water-energy nexus. Second, is the availability of capital for business creation in developing countries. Third, are examples of technology development that suggest that nonconventional approaches to treat water and wastewater are in existence and could be refined to meet the conditions of a “new paradigm.”
The Water-Energy Nexus
Energy and water use are intimately linked. Domestic water use requires significant energy for pumping and treatment. American public water supply and treatment facilities consume about 56 billion kilowatt-hours per year—enough electricity to power more than 5 million homes for an entire year ( EPA, 2009 ). Thus, saving water saves energy and results in fewer greenhouse gas emissions. On the other hand, energy production uses and impacts domestic water supplies. As stated previously, cooling water represents nearly half of the volumetric water use in the United States annually. The power industry requires reliable supplies of water for cooling, for flue gas desulfurization and ash handling, and for hydroelectric power generation. As population grows, so does the demand for electric power and water for agricultural, municipal, residential, commercial, industrial, and power generation uses, potentially straining water supplies. Because the water supply is limited, growth in demand can only be met by developing technologies that reduce the volume of water required per kilowatt-hour of power generated. In short, the ideal way to reduce water consumption is to increase energy efficiency.
Rapidly developing nations must be able to realize the benefits of energy efficiency while building their infrastructure. A study by the McKinsey Global Institute (2007) reported that the global demand for energy is estimated to grow at a rate averaging 2.2 percent a year up to the year 2020, a rate that is the fastest since 1986. Developing countries will account for an overwhelming 85 percent of energy demand growth to the year 2020. However, the McKinsey study reported that it is possible to improve energy efficiency in a manner that could cut energy demand growth by at least half. The greatest productivity improvement opportunity is in the global residential sector (the world’s largest consumer of energy). Bringing the preferred existing, or yet to be discovered, ways to increase energy efficiency in buildings, residences, and other operations that derive electricity from stationary power sources is essential to mitigate water stress and decrease water use.
The water-energy nexus extends beyond efficiency. Today, a transition in energy production is occurring for a model of distributed power systems ( Platz and Schroeder, 2007 ). While most of the world’s power produced today comes from centralized power plants using fossil fuel combustion or nuclear fission to drive steam turbines, these centralized power systems require significant capital investments and extensive distribution networks to reach consumers. The development of small-scale, distributed energy systems is an area of research and development today. This includes solar generation, wind and water turbine generators, and other technologies that create electricity at the point of demand. As markets increase, the cost of onsite power generation will decrease due to economies of scale and it should be possible to infuse developing countries with power generation without the 10 to 20 years of construction of conventional stationary power systems. With the advent of distributed energy comes the potential for distributed water and wastewater treatment, using technologies or approaches that differ significantly from conventional water and wastewater systems. In fact, it is possible to create wastewater treatment systems that generate electricity that would themselves be a distributed power generator.
Innovative Financing and Business Models for the Water, Sanitation, and Energy Sectors
Intermediate solutions that address the technical and financial gap between point-of-use technologies and the heavy infrastructure projects require innovative financing approaches. National governments often lack the financial means to extend water, sanitation, and energy coverage through infrastructure investments. Cardone and Fonseca (2006) describe innovative financing trends and case studies for small-town water and sanitation services.
In most developing countries, financial services such as bank loans, insurance, and pension funds are not readily accessible by the poor ( NWP, 2007 ). Microfinancing services supply capital to poor people considered “unbankable” by the conventional financial sector, with loans for as little as $50 ( Morgan Stanley, 2007 ). Since 1976, microfinancing mechanisms have been providing small loans to the poor and the microfinance sector has grown significantly despite the absence of specific financial sector policies.
Microfinancial institutions (MFIs) differ from traditional banking institutions in several ways ( J. P. Morgan, 2009 ). MFIs emphasize both their financial profitability and their social impact (i.e., double bottom line). MFIs also have higher net interest rate margins compared to commercial banks. This is due to the relatively high interest rates charged to microfinance clients typically resulting from higher administrative expenses because of the location of clients, small transaction size, and frequent interaction with MFI staff. In 2006, the average worldwide microfinance lending rate stood at 24.8 percent ( J. P. Morgan, 2009 ). Furthermore, MFIs have typically had a stronger asset quality than mainstream banks in emerging markets, due to a good knowledge of customers and strong incentives for clients to pay and establish a good credit history ( J. P. Morgan, 2009 ).
Microfinancing has typically not been available for financing water supply and sanitation activities primarily because of a lack of awareness of the business case for water and sanitation projects. Water and sanitation projects become bankable when assets such as pumps, turbines, and solar panels are introduced. These assets provide collateral and the means for generating recurring income streams and fee-based services. Emphasized investment in distributed energy technologies for the poor combined with local currency lending mechanisms may flourish as the microfinancing markets mature and infrastructure spending increases.
Microfinancing mechanisms can serve those at the household and small community levels. Platz and Schroeder (2007) provided case studies in Africa and Latin America that describe how larger scale programs can be financed and implemented. The delivery of water and electricity to the poor is characterized by low levels of cost recovery and requires long-term financial investments. Full cost recovery in the least developed countries can be problematic, and targeted subsidies often are needed to finance large projects.
Although the current financial crisis is not expected to affect long-term investment in energy infrastructure projects, delays in bringing current projects to completion are expected ( IEA, 2008 ). Privatization projects in the water and sanitation sector have largely failed ( Bayliss and McKinley, 2007 ), and global private water investments in 2007 were low, at just $3.2 billion ( World Bank, 2008 ). Substantially more funding is needed to strengthen public sector services, and clean renewable technologies have the potential to attract new significant investment while offering a return on investment and societal benefits. Bringing these opportunities to fruition requires strong collaboration between the engineering, financial, and health sectors to ensure community acceptance and economic sustainability.
New Water and Sanitation Technologies
As was discussed previously, most research in the United States and elsewhere has focused on improving conventional infrastructure. In some cases, the impediments to improve existing technology have resulted in the development of new approaches to replace older technology. Examples of this, which have applications in developing countries, are membrane separations and UV disinfection. Membrane processes are advanced filters that are capable of removing particulate and, in some cases, dissolved contaminants of water. They are effective in the removal of bacteria and viruses and can also remove other contaminants depending on the fabrication of the membrane itself. Much of the most recent research has focused on reducing the pressure needed to drive water through the membrane, and considerable progress has been made in this area. Decreasing pressure results in lower energy needs and should allow for membrane treatment using some of the distributed power systems discussed previously. Small-scale household or community production of water for drinking and body contact using coupled energy production-membrane filtration units may represent an area of study.
UV disinfection has been an area of research to replace existing disinfection strategies (e.g., chlorination) that result in the formation of unwanted disinfection byproducts. Classic UV disinfection uses mercury vapor lamps, which are not likely to be used in the developing world due to cost, poor durability, energy consumption, and the potential effects of mercury wastes. Considerable technology development has been occurring in the area of light emitting diodes and other “lamps” that are far less energy consuming than traditional light bulbs. They also offer increased durability and longevity. The development of low power lamps that produce UV in a spectrum similar to a mercury vapor lamp would be ideal for use in the developing world to disinfect water, again coupled with a distributed energy source.
Microbial Fuel Cells
One promising technology for the treatment of human and animal wastes is microbial fuel cells (MFCs). While simple in design, MFCs harness the natural ability of bacteria to break down organic matter and create electricity directly. MFCs function with bacteria to oxidize organic matter (i.e., the electron donor) on the anode under anoxic conditions and transfer the electrons to a cathode through a wire. Oxidation of these recently fixed sources of organic carbon does not contribute net carbon dioxide to the atmosphere and, unlike hydrogen fuel cells, there is no need for extensive preprocessing of the fuel or for expensive catalysts ( Lovley, 2006 ). By converting biochemical to electrical energy, their most likely near-term application is as a method of producing energy from wastewater ( Logan and Regan, 2006 ). What currently is not known is how best to integrate MFC approaches in systems such as latrines or small-scale waste facilities. Electricity production from waste is an interesting possibility that may create a market for sanitation that currently is not in existence.
Geographic Information Sciences for Decision Makers
Geographic information sciences (GIS) combine remote sensing, geographic information systems, cartography, and surveying, interrelating with mathematics, and the physical, biological, and social sciences. It empowers researchers and decision makers to evaluate complex environmental systems and the interconnections with human health. New tools for modeling, predicting, and forecasting the water resources sustainability, quantity, and quality are being used in developing countries. The use of novel sensors, wireless and broadband technologies, high-performance computing, and real-time data assimilation is being promoted with the objective of better understanding the Earth’s water resources and related biogeochemical cycles; this goal could lead to better management of activities that impact human health ( Schnoor, 2008 ). Multidisciplinary innovations such as these provide a foundation for better decision making on global issues of water quality, water resources, and sanitation.
Nanomaterials Applications of nanoscience for water treatment are in the market and expanding (Hillie et al., 2007). They include
- nanofiltration membranes for removal of salts and micropollutants, and for wastewater treatment;
- use of Attapulgite clay, zeolite, and polymer filters, which can be manipulated on the nanoscale for greater control over pore size of filter membranes;
- nanocatalysts and magnetic nanoparticles that will enable the use of heavily polluted water for drinking, sanitation, and irrigation; and
- nanosensors for detection of chemical and biochemical contaminants.
The impact of nanotechnologies on human health is a growing research need. Risks and social issues associated with promoting advanced filtration techniques in developing countries need to be understood and communicated to promote transparency and adoption of the technologies.
Research Needs for a New Water and Sanitation Infrastructure Model
The development of a new water and sanitation infrastructure model requires multidisciplinary, systems-based thinking and innovations that vary from successful approaches adopted by developed nations. Advances and improvements in the necessary disciplines are occurring on a global scale; integrating the health, engineering, economic, political, technological, and social aspects for sustainable solutions for the world’s poor calls for research in the following subjects:
- Development of robust and appropriate water technologies to create safe drinking water;
- Development of robust and appropriate wastewater and dry sanitation technologies;
- Technology transfer and development of markets for these technologies;
- Development of best practices in watershed protection, water conservation, and sustainable energy systems for rural and urban populations;
- Tailoring water and sanitation for microfinanced enterprises and investment banking institutions;
- Scalability of innovative technologies for maximum impact;
- Health and epidemiological concerns of a successful implementation; and
- Outreach and education to improve transparency at all levels of government.
IMPROVING URBAN WATER AND SANITATION SERVICES: HEALTH, ACCESS, AND BOUNDARIES 6
Kristof Bostoen, Ph.D., M.Sc.
London School of Hygiene and Tropical Medicine
Pete Kolsky, Ph.D.
The World Bank
Caroline Hunt, Ph.D.
Those who live in cities depend upon resources from outside city boundaries. Use of external water resources is one of the important ways a city affects, and is affected by, its surroundings. During rapid urban growth, these interactions become increasingly important for both the city and its environment.
Water flows back and forth between the natural environment and the urban community ( Figure 4-7 ). Water supply brings water from the broader environment into the community, while drainage and sewerage returns it to the ‘natural’ environment. Water in such transfers is never pure H 2 O, but is always mixed with other matter, as illustrated in Figure 4-7 . Often this ‘other matter’ includes pathogens (disease-causing organisms).
The water balance.
Whatever water comes into a community has to be returned to the natural environment. Even with recycling and storage, the outflow must more or less equal the inflow, or else flooding will occur. Despite this fairly obvious fact, efforts are frequently made to improve community water supply without improving drainage. If water is returned to the natural environment with chemical or biological pollution, the contamination does not always disappear or die off, but can return to threaten the health of the polluting community or that of one downstream.
This chapter examines issues related to water supply and sanitation services, which are of particular relevance to low-income communities. The second section of this chapter looks at health issues relating to water, while the third section examines the targets set by the international community for water and sanitation and the challenges regarding the achievements of these targets.
As problems of water shortages and pollution are transferred from the local to the broader environment, the challenge shifts from one of maintaining human health to one of preserving the integrity of life-support systems for future generations ( McGranahan et al, 2001 ). These transitions are well known and documented with regards to the water cycle.
The water cycle within the urban area, as illustrated in Figure 4-7 , occurs at each spatial scale of the city; for a given neighbourhood, the rest of the city is seen as the broader environment. These different subdivisions, or boundaries, often create institutional issues which in turn have an impact upon service quality and health; these are explored in the fourth section of the chapter.
How Water Supply, Sanitation and Hygiene Affect Health
Below, in the following two sub-sections is a description of two common models to describe the relationships between water supply, sanitation, hygiene and human health, as they are understood at present. The third sub-section refers to recent and forthcoming research in this field.
Classifications of Water-Related Infections
The first model has evolved from earlier work, grouping water-related infectious diseases by broad routes of transmission ( Feachem et al, 1977 ; White et al, 1972 ). The categories are defined by the types of intervention that can control morbidity and mortality, rather than by the biological taxonomy of the organisms that cause them. As such, this model has helped engineers and public health professionals to work together on practical control strategies ( Kolsky, 1993 ). A similar classification exists for excreta-related diseases ( Feachem et al, 1983a ) but has been less widely used. There are four categories in the Bradley-Feachem classification of water-related disease:
- Faecal-oral (waterborne and waterwashed). These include infections that are transmitted by swallowing faecally contaminated matter (food and water) containing pathogens. They can be caused by lack of sufficient water to maintain personal and domestic hygiene as well as by drinking contaminated water. Diseases in this group include, among others, diarrhoeal diseases, typhoid, cholera and hepatitis A and E.
- Strictly water-washed (skin and eye infections). These are conditions that are exacerbated by lack of water for washing and hygiene, but are not faecal-oral. These diseases are largely related to skin and eyes, such as scabies, trachoma and conjunctivitis.
- Water-based aquatic intermediate host . Aquatic organisms such as snails act as hosts to parasites, which then infect humans either by being swallowed or through contact in water (e.g. by piercing the skin of those wading in the water). Diseases in this group include guinea worm and schistosomiasis.
- Water-related insect vector . These diseases depend on insect vectors, such as mosquitoes and flies, which breed in or near water. They transmit disease to humans, for example, through bites. The diseases involved include malaria, filariasis, yellow fever, dengue and onchocerciasis (river blindness).
From the four categories in the Bradley-Feachem classification it becomes clear that interventions focused on water quantity have broader impact than those focused on water quality. Water quality only affects faecal-oral diseases, whereas quantity affects both faecal-oral and water washed diseases. The relative importance of water quantity and its quality will be discussed later in this chapter.
Diarrhoeal diseases, which are faecal-oral, are responsible for the greatest number of episodes of illness (morbidity) and deaths (mortality) worldwide, compared to any other single classification of water and sanitation-related disease. This is shown in Table 4-6 , based on data presented for World Health Organization (WHO) member states. It has been estimated that diarrhoeal disease represents 90 per cent of the health impact associated with water supply and sanitation ( White et al, 1972 ). Diarrhoeal diseases are estimated to kill around 1.8 million people every year worldwide ( WHO, 2004 ) of which the overwhelming majority is children. This toll is equivalent to 12 jumbo jet crashes every day or almost twice (1.9) the number of people who ‘died in the World Trade Center on the 11th of September 2001’ per day. There is some reason to believe that the number of deaths has fallen since the 1980s, possibly due to water and sanitation programmes and increased use of oral rehydration therapy ( Bern et al, 1992 ). However, it appears that the number of episodes of diarrhoeal disease has remained constant.
Health Impacts of Water- and Sanitation-Related Diseases.
Approximately 90 per cent of diarrhoeal disease cases are estimated to be attributable to environmental factors ( Murray and Lopez, 1996 ). Apart from water supply, sanitation and hygiene, diarrhoeal disease is also associated with a number of other risk factors including age, malnutrition, lack of breastfeeding, and seasonality.
The F-Diagram
A second model is the F-diagram, depicted by Wagner and Lanoix (1958) ( Figure 4-8 ), which has been widely used as a model of faecal-oral disease transmission. Unless faeces are isolated from potential contact with humans, animals and insects, pathogens may be carried on unwashed hands, in contaminated water or food, or via flies and other insects on to further human hosts. The first way to stop or reduce transmission is to ensure the safe disposal of faeces, through sanitation. Safe excreta disposal and washing hands following defecation is referred to as ‘the first barrier’ and considered the most important health intervention, as it keeps faecal pathogens out of the living environment. Children’s faeces in particular are known to contain a high load of pathogenic organisms, such as Ascaris and Trichuris, but are also least likely to be safely disposed of ( Cairncross, 1989 ; Kolsky, 1993 ). The secondary barriers to faecal-oral disease transmission protect people from whatever faecal contamination of the environment is present. These are based on hygienic practices, such as washing hands before handling food, fly control, safe food storage and the use of footwear.
F-diagram. SOURCE: After Wagner and Lanoix (1958). With permission from the World Health Organization.
The F-diagram graphically presents multiple routes of transmission. A single type of pathogen may be transmitted by several of these routes, and the population at risk may be vulnerable to many different pathogens, which may favour different routes. Numerous commentators have advocated integrated measures to control diarrhoeal disease by combating multiple routes of transmission ( Lewin et al, 1996 ; Curtis et al, 2000a ). The greater the range of interventions, the greater the chance in successfully reducing diarrhoeal disease transmission. The F-diagram also shows that while water quality only affects one route, the quantity of water available for personal and domestic hygiene affects almost all routes.
Following the discovery in the 19th century of the undeniable role that water quality played in the epidemics of cholera and typhoid, there was a natural focus on the improvement of drinking water quality. This focus produced dramatic results in the reduction of waterborne epidemics. The F-diagram clearly shows that this would be the case where water contamination is the main route of transmission.
Where routes other than water consumption are more important for disease transmission, however, improving water quality will have far less effect. While waterborne epidemics are dramatic and alarming surprises, the sad truth is that the everyday endemic (non-epidemic) toll of faecal-oral disease is far, far higher, and most of the latter seems to be transmitted through routes other than water. While improving water quality does not necessarily affect endemic transmission, increasing the quantities of water available to improve personal and domestic hygiene can have a greater effect on this unacceptable toll ( Cairncross, 1995 ). Most health benefits will be obtained from large amounts of water of a good quality. But if resources are scarce, public health professionals generally recognize the greater importance of access to water in quantity for hygiene, compared with the quality of that water ( Esrey et al, 1985 , 1991 ). Unfortunately, in practice, the main efforts in ‘water and sanitation for low-income areas’ are often still directed towards the improvement of the water quality of the public water supply, rather than improving access by poor households, and thus the quantity of water that those households can use. The beneficiaries of such efforts are more likely to be people who already have access to water than people with no access. Sanitation and hygiene promotion are even lower priorities in practice, although the principles of the F-diagram suggest that they should have equal or higher priority ( Curtis et al, 2000b ).
Recent and Forthcoming Research
Most studies of the impact of water quality have been based upon water quality measurements at the source or collection point. It is known however, that the degree of faecal contamination of water increases during transport to the household ( Clasen and Bastable, 2003 ). There is also increasing evidence that improving water quality at the point of use has a positive health impact ( Conroy et al, 2001 ; Iijima et al, 2001 ; Fewtrell et al, 2005 ; Clasen, 2006 ). This is regarded by some as an exception to the dominant paradigm ( Clasen and Cairncross, 2004 ). While data support health benefits for people that have at least 15 litres of water per capita per day there are reasons to believe that benefits are reduced when access levels to water are lower ( Clasen, 2006 ). However, at this time not enough data are available to substantiate this ( Clasen, 2006 ). Systematic reviews and meta-analyses, such as those of the Cochrane Library infectious diseases group ( Clasen et al, 2004 ) and field research will be needed to clarify these new findings and examine if these are in conflict with the current paradigm.
What Does All This Mean?
These models clarify the complex relationships between water, sanitation, hygiene behaviour and health. For example, good hygiene is more important in low-income areas where environmental exposure to pathogens is greater; residents of relatively clean areas can (and often do!) get away with lower standards of hygiene. Those who practise poor hygiene are certainly at greater risk than those who practise good hygiene, even in relatively clean environments. Water quantity is generally more important than water quality, because increased quantities of water promote good hygiene, and can prevent faecal-oral transmission by a number of different routes; increased quantities of water also reduce skin and eye infections. Only when drinking water is the main source of infection will water quality be more important than quantity. This is rarely the case where diarrhoea is endemic.
This means that in most cases within an urban setting, water distribution (access to water in quantity) is more important than public water treatment (its quality) until a certain relatively high level of environmental hygiene has been reached. Water treatment at the point of use seems to give health benefits, but it is not clear if a certain level of access to water is required to profit from such an approach. Finally, the quantity of water which people can actually use is vitally dependent upon access, as shown by Figure 4-10 ; the issue of access will be further explored in the following section.
FIGURE 4-10
Relation between water consumption and time involved in water collection. SOURCE: Reprinted from Cairncross and Feachem (1993) with permission from John Wiley (more...)
Access to Improved Sanitation and Water Sources
‘Water for life’, the 2005 global water and sanitation assessment, contains the most up-to-date coverage data for most of the countries in the world ( WHO/UNICEF, 2005 ). Since the Global Assessment 2000 Report (GA2000) ( WHO/UNICEF, 2000 ) the United Nations (UN) Joint Monitoring Programme (JMP) does not report on ‘safe’ drinking water and ‘adequate’ sanitation. Instead, access to ‘improved’ water supply and sanitation technology types are now reported (see Table 4-7 ). This change in terminology reflects both past misrepresentation, and future uncertainty, in judging and defining services as safe in terms of human health. According to the report, over 2.6 billion people worldwide are without access to improved sanitation and over 1.1 billion do not have access to improved water supply. While many people have gained access since 2000, the number without access has remained the same ( WHO/UNICEF, 2006 ).
Water Supply and Sanitation Technologies Considered to Be Improved and Unimproved in WHO/UNICEF Global Assessment 2000.
Asia and Africa have the lowest levels of service coverage. In Asia, less than half the region’s population have access to adequate sanitation. When comparing individual countries, the African region has the highest proportion of countries with less than 50 per cent water supply and sanitation coverage. In all regions, apart from North America, rural coverage is lower than urban coverage for both water supply and sanitation.
The Global Assessment 2000 presented the status of the sector using consumer-based data for the first time. These data were drawn from large nationally representative household sample surveys, such as the United States Agency for International Development’s (USAID) Demographic and Health Survey (DHS) and United Nations Children’s Fund’s (UNICEF) Multiple Indicator Cluster Survey (MICS) and national census data. The GA 2000 thus presented a better baseline for future targets than previous reports. The WHO/UNICEF Joint Monitoring Programme for Water Supply and Sanitation (JMP), which published the GA 2000, continues to update these data to monitor progress to the Millennium Development Goal Target for water and sanitation. The latest report to date is Meeting the MDG Drinking Water and Sanitation Target ( WHO/UNICEF, 2006 ), which is available together with current data at the JMP website ( www.wssinfo.org ).
Targets For the Future
The UN Millennium Summit adopted the target of halving the proportion of people who are unable to reach, or to afford safe drinking water by the year 2015. The 2002 UN World Summit on Sustainable Development (WSSD) in Johannesburg has adopted the same target for access to sanitation facilities and the application of hygiene practices.
The compilation of the GA2000 has greatly improved data quality, using survey data. However, there is still a need to standardize survey outcomes to make results comparable. Figure 4-9 shows the typical scatter of results of the different surveys over the last 20 years for access to improved water in urban Niger. The variation in results is less for urban than for rural areas. Variations between different surveys are also less for household connections than for other improved access, which is probably a reflection of the interest of the water utility in keeping track of its customers and the ease of defining this way of delivering water to households.
Variation on access in various surveys. NOTE: HC = household connections. SOURCE: UNICEF. NOTE: The original source of this figure is http://www.childinfo.org/files/NER_wat.pdf.
Urban populations in Asia and Africa are predicted to almost double over the next 30 years. Against this trend, meeting the International Development Target of halving the proportion of those unserved by water by 2015 would mean providing water services to more than 300,000 additional people every day over the next 15 years. Halving the number unserved by sanitation requires provision of services to over 400,000 additional people per day.
Limitations to the Use of Routine Data Sources
The use of existing surveys such as the DHS, MICS and national censuses, as in the GA 2000, has the advantage of being cost efficient, but it also has its drawbacks. These surveys have usually been designed to give a picture of ‘the average household’ on a national level and sometimes on a regional scale. Designed for other purposes, they cannot provide sector-specific (water, sanitation and hygiene) data at a local level that can be used for project implementation, evaluation and local decision making.
Large surveys such as the DHS require major administrative and organizational work, which means that they are unlikely to take place in countries or areas experiencing conflict or natural disasters.
To achieve better measurements in the field of water, sanitation and health practices there is a need for a simple standardized sample technique and a standardized set of indicators. Moreover, not only the collection techniques, but the interpretation and the type and extent of analyses need to be agreed upon if results are to be compared worldwide.
Access to Improved Services and Its Relation with Health
The level and type of service both have the potential to influence health. However, numerous other factors, which influence the use and nature of the service, also affect health risk, in some cases to a greater extent than the level or type of service itself. These factors include: access to, and use of services; system maintenance; treatment; seasonality; water sources; and pathogenspecific factors. Poverty is very often a key variable behind many of the factors listed above, most notably access to services.
The improvement of water supply and sanitation has attracted particular interest in reducing diarrhoeal disease ( Feachem et al, 1983b ; Esrey et al, 1985 ). These environmental improvements, together with improvements in living standards, played a major role in reducing diarrhoea rates and controlling endemic typhoid and cholera in Europe and North America between 1860 and 1940 ( Esrey et al, 1985 ). Similar effects were anticipated from equivalent improvements in low income countries, and these expectations contributed to the declaration of the Water Decade. In 1977, the UN Water Conference at Mar del Plata set up an ‘International Drinking Water Supply and Sanitation Decade’ for 1981–1990. Its aim was to make access to clean drinking water available across the world.
Although improving access to water and sanitation projects improves health ( Feachem et al, 1983b ; Esrey et al, 1985 ), it is difficult to link these achieved health benefits back to specific improvements. Many attempts have been made to measure the health impact from water supply, sanitation and, more recently, hygiene practices. Even attempts under the supervision of eminent specialists to measure the health impacts of water supplies and sanitation have produced almost useless or meaningless results ( Cairncross, 1999 ). Health impact studies are, for that reason, not an operational tool for project evaluation or ‘fine tuning’ of interventions ( Cairncross, 1990 ). This led various organizations like the WHO and the World Bank to adopt the Minimum Evaluation Procedure ( WHO, 1983 ), which concentrates on measuring functioning and use of services rather than measuring their health impacts ( World Bank, 1976 ; Briscoe et al, 1985 ; Esrey et al, 1985 ; Cairncross, 1999 ).
While the health benefits of increased water quantity are known, they are difficult to measure and to attribute back to increases in supply. There is, for example, a clear but counterintuitive relation between the time needed to collect water and the amount of water collected ( Figure 4-10 ). It is known that an increase in water consumption increases the water used for hygiene, which improves health. So, one might expect that reducing the time it takes to secure daily supplies to below 30 minutes would have a beneficial health impact. However, a reduction of the collection time of between 30 and 3 minutes will actually have little impact. Those who spend less than 3 minutes for water collection usually have a house-hold connection. Note also that collection time includes queuing time, which can be significant in areas with relatively closely spaced taps with intermittent service, or in areas that are serving large populations. In various parts of Africa, reports show that while distance to source diminished or stayed the same, collection times for water increased ( Thompson et al, 2002 ; UN-Habitat, 2003 ).
While the main ways water, sanitation and health practices relate to health are broadly understood in theory, their real-world interactions are far more complex. However, increasing access to water and sanitation is clearly recognized as leading to health benefits, and new international targets have been set for that reason. Although improved access might improve health, it is methodologically extremely difficult to attribute improvements in health exclusively to specific interventions, on a project-by-project basis. This makes health impact an unsuitable outcome measure for project evaluation. The current indicators based on level and type of service also have their limitations. Better sector specific indicators and survey tools need to be developed.
Boundary Issues and the Urban Environment
Differing perspectives.
Figure 4-11 shows the urban environment from the point of view of the householder. The home is at the centre, and is the householder’s first priority for environmental management. If the householder is able to maintain the home in a relatively clean and pleasant condition, the next priority becomes the surrounding street (peri-domestic). Local and informal lane arrangements may, for example, develop among neighbourhoods to ensure that rubbish does not pile up in the street, or clog drains. If the home and street are relatively clean, then some citizens will be concerned about the state of the environment in their larger neighbourhood (ward); when these problems are addressed, attention can then be focused on the rest of the city, and eventually, on the environment outside the city. It is natural that the focus of environmental concern spreads further outwards as the problems at each of the smaller (inner) scales are resolved.
FIGURE 4-11
Scales of the urban environment, as seen by a householder. SOURCE: Kolsky (1996) unpublished lecture notes, London School of Hygiene and Tropical Medicine.
It turns out that the householder’s perspective and priorities are similar to those that emerge from a public health perspective. As most of the victims of poor environmental health are children under five, it makes sense to focus attention on where they spend the most time, which is at home. We have also seen that water access at the household scale is critical to increasing the quantity of water used to improve hygiene. The construction of public toilets half a kilometre from the house may offer limited improvement in convenience and dignity of some adults, but will not significantly improve the health of children in the community, who will rarely if ever use such services. Improving the quality of river water by controlling the quality of the wastewater discharge may be of ecological benefit, but it makes no difference to a household’s health unless that improvement is translated into improved drinking water quality at the household scale. The public health priority for environmental improvement thus becomes the household, followed by its immediate neighbourhood.
These differing scales of the urban environment are reflected in the structure of environmental service provision. Water supply, sewerage, storm drainage and solid waste management all involve the flow of mass between the individual household and the larger environment. Figure 4-12 shows the superimposition of a water supply system upon the scales of the environment shown in Figure 4-11 , and similar sketches can be prepared for the other environmental services such as sewage disposal and solid waste management. Table 4-8 also shows the physical infrastructure associated with each level of aggregation.
FIGURE 4-12
Scales of water supply infrastructure matched to the urban environment. SOURCE: Kolsky (2006) unpublished lecture notes, London School of Hygiene and Tropical Medicine.
Scales of the Urban Environment and Water, Sewerage and Drainage and Solid Waste-Related Infrastructure Issues.
The perspective of the service provider (e.g. the sewerage utility, the water supply engineer, and so on) is often different from that of the householder and public health specialist. They will look at the same system, but usually focus on different concerns, as shown in Figure 4-13 . The highest priority of the water engineer is often the intake and central treatment works. Their attitude is, if this link of the chain fails, all the rest will fail.
FIGURE 4-13
Water supply infrastructure and priorities, as seen by technical professionals. SOURCE: Kolsky (2006) unpublished lecture notes, London School of Hygiene and Tropical Medicine.
Construction of centralized works often involves substantial amounts of financial capital and technical sophistication, both of which contribute to the professional standing of the individuals involved. Primary mains are the second priority after the central works, because of the relatively large impact of the failure or inadequacy of these links in the chain. While central pipes are more expensive per metre, the majority of the cost in the distribution system is tied up in the large number of small outlying lines. Individual street mains and house connections are often at the periphery of the technical professional’s vision. Indeed, in many cases the ‘outermost rings’ of the technical professional are virtually ignored; water is provided to a public tap to serve a neighbourhood, and what happens to the water after that is not the practical concern of the utility. This focus on centralized works is even less appropriate for sanitation infrastructure, where large amounts of resources may easily be spent upon centralized waste treatment works of marginal public health benefit in comparison with the provision of basic household access.
Unfortunately, the poor live in the outermost ‘marginal’ rings. These ‘rings’ often represent very clearly definable neighbourhoods which are rarely laid out for service provision, have dubious tenure relationships, and are thus not a priority for service delivery. They are therefore easily excluded, yet it is here where the battle for urban public health is won or lost.
Boundaries and Their Impact on Services
We define a boundary as the limit beyond which an individual or group feels no responsibility. Boundaries can be marked by physical, legal, bureaucratic, psychological or customary limits. They can include formal district boundaries in rural areas, and ward boundaries in urban areas; they may also be as informal, but also as strong, as the sense of community around a courtyard or square.
The use of a subjective word like ‘feels’ in the definition seems odd, but the reason for its use is a pragmatic one. The world is filled with legal or administrative boundaries without practical meaning because those within the boundary do not feel, directly or indirectly, the consequences of their actions.
Boundaries permit the breakdown of complex problems into simpler parts, and the corresponding delegation of responsibilities and tasks. By limiting responsibilities, they become manageable, both for the person delegating and for the person performing the task. It is difficult to be responsible for everything, but we can accept responsibility within given boundaries.
Classification of Boundary Problems
While boundaries are a useful political, social and administrative device, they are not without drawbacks. There are, at least, four related categories of problems arising from boundaries.
Complete externalities When the damage of my actions to others does not affect me, I have no incentive to change my behaviour. This situation is known to economists as an ‘externality’, and in this case, explains why wastewater treatment always lags behind drinking water as a community priority. Drinking water affects the members of a community directly, so it is in their interest to take appropriate action to ensure its quality. Sewage effluent quality affects only downstream users; upstream community members causing the problem have no direct interest in resolving it for their downstream neighbours. Government action is often required to solve such problems. Although environmental pollution is the classic example of such a boundary problem, there are also administrative examples of externalities, as described below.
Partial externalities In some cases, I do care about the damage I cause outside my boundaries, but if most of that damage will occur in any event, why should I change my behaviour? Hardin describes this in his classic paper The Tragedy of the Commons ( Hardin, 1968 ). The paper examines the behaviour of individual shepherds responsible for grazing on common land. Collectively, it is clear that they would be better off with fewer sheep grazing on the commons, because in the long term, overgrazing will destroy the resource. Individually, however, each shepherd is better off grazing as many of his sheep on the land as possible; after all, if he restrains his behaviour, the commons will still be destroyed by others, and he will have sacrificed in vain. Some form of social arrangement needs to be worked out, or else the commons will be finished. The difficulty of working out such social arrangements, complete with effective sanctions against those who violate them, lies at the heart of many environmental and social problems. Rubbish dumping in urban slums often falls within this category.
Badly drawn boundaries In some cases, things could work better if boundaries were simply redrawn. Engineers often refer to drainage networks and other urban infrastructure as trees. Such trees have large ‘trunk’ mains, and smaller ‘branch’ lines, and the image of a tree effectively conveys the notion of many smaller entities combining into a larger one. Most of the length of a drainage network, and most of the cost, lies in the many small branches rather than in the trunk line. While trunk lines are certainly more expensive per metre, the total cost of a network is dominated by the smaller branches at a smaller unit cost making up the outer branches. Such lines are often technically simple and individually not expensive; added up they represent the main cost of the network.
Traditionally, centralized authorities have taken responsibility for entire drainage networks on the grounds that all drains up to the individual property boundaries are public goods. This model was developed in the industrialized world and has served quite well there. Alternative approaches, however, have emerged in the cities of the developing world. Municipal authorities there are devolving the responsibility for these smaller lines to local community groups or non-governmental organizations (NGOs). This can be done because small branches are technically simple, and can be managed better by closer supervision within the community than by the distant municipal authorities. This does not mean that central authority should disengage totally in regards to compliance and appropriateness of these activities.
Redrawing boundaries in the water sector is not a new idea; the regional water authorities in Britain ( Okun, 1978 ) are another practical demonstration of the benefits of more rational boundary definition. The environmental economics literature ( Ruff, 1970 ) stresses the need to draw boundaries so that benefits and costs of activities are felt by those who take the action, thus reducing the boundary problem of externalities.
The category of badly drawn boundaries includes also cases where there are gaps between boundaries (so no group feels responsible), and cases where boundaries overlap (where more groups claim the same responsibility or authority).
Boundaries as barriers Movement of ideas and resources across boundaries is always more difficult than within boundaries. For example, at the start of the International Drinking Water Supply and Sanitation Decade, a need was recognized to integrate the services of water supply and ‘sanitation’, used here as a euphemism for excreta disposal. This made sense both in conceptual public health terms (as both are involved in the spread of faecal-oral disease) and in practical engineering terms where water-based sewerage is the main means of excreta disposal, and thus depends directly on the water supply. Communications between these services improved as intended, under the new boundaries.
Redrawing the boundaries around water supply and excreta disposal, however, caused the nominal separation of sanitary sewerage and surface water drainage even where these systems are physically interconnected. In the same street separate crews clean these ‘separate’ drains, requiring separate transport and equipment at different times. Because of the new administrative boundaries, these crews no longer communicate, and no longer share access to the same resources.
Other types of boundary create other communications problems in water, sanitation and other sectors. Because external support agencies do not wish to become entangled in an open-yended [ sic ] commitment to paying recurrent costs, they have traditionally limited their involvement to capital investment. This means that both international and local resources are drawn to the investment sector, to the neglect of the operational aspects. Recently created municipal development authorities created in recent decades are responsible for municipal investment, but not for the day-to-day maintenance of the infrastructure they develop. There is often a strong feeling among those running the infrastructure that those planning and designing it don’t understand basic operational reality.
Boundaries define the problem considered by those with responsibilities within these same boundaries. There is, for example, an administrative boundary between street sweeping and drain maintenance. The street sweeper’s boundary extends only to keeping the street clean, so sweeping sediment and debris into a drain is seen as perfectly acceptable. Similarly, the drain cleaner’s boundary extends only to keeping the drain clean, so that emptying debris onto the street for subsequent pick-up by the solid waste department is also seen as acceptable. If the street sweeper returns before the solid waste department picks up the debris, then it will be swept back into the drain, thus achieving the environmental goal of perfect recycling! In this case, the real issue is ‘solid waste management’ which cuts across the pre-existing boundaries of street sweepers and drain cleaners.
Public and Private Domains as a Special Example of Boundary Problems
For a long time public health engineering has been focused on public domains to bring health improvements to deprived populations. This has involved construction of large-scale urban water and sanitation infrastructure. A spatial model of disease transmission in public and private domains illustrates the move away from this traditional, engineering approach to public health ( Cairncross et al, 1996 ). Diseases transmitted on the household level have to be dealt with via interventions that reach the household level. This puts more emphasis on private health at household level and the need to understand decisions made and actions taken at household level and their relation to environmental health. The private domain is distinctly different from the public domain in which the intervention of a public authority is required to prevent disease transmission. Some of these spatial problems relating to this model have been discussed in the paragraphs above. This model acknowledges the importance of household practices and behaviour without ignoring the public domain.
Many studies have investigated the links between public water supplies and household contamination of stored water ( Kirchhoff et al, 1985 ; Deb et al, 1986 ; Jonnalagadda and Bhat, 1995 ; Mintz et al, 1995 ; Jagals et al, 1997 ; Quick et al, 1999 ). Findings have been rather mixed. There is a growing consensus that diarrhoeal disease pathogens originating within the home, as found in household water storage vessels, are less of a threat to household health than pathogens found in source water supplies (e.g. from public wells) ( VanDerslice and Briscoe, 1993 ). There appears to be degrees of immunity to pathogens commonly found within the household. These complexities are acknowledged and explored within the public-private domain model. The recent literature on point-of-use treatment of drinking water ( Conroy et al, 2001 ; Hellard et al, 2001 ; Iijima 2001 ; Fewtrell et al, 2005 ; Clasen, 2006 ) seems to indicate that there are health benefits from improving drinking water quality at the household level. Further research will be required to determine if this is true for households with limited access to water.
Several studies have looked at the impact of interventions at household scale when the neighbourhood is contaminated with faeces ( Cairncross et al, 1996 ). Others have logically argued that interventions should be targeted at those most in need. It has been suggested that children who are not breast-fed are more susceptible to diarrhoeal disease and as such may benefit more from water supply and sanitation interventions than breast-fed infants ( Esrey et al, 1985 , 1991 ). The logistical difficulties in carrying out this approach may, however, make it impracticable.
Water Stress at Global and Household Scales, a Boundary Point of View
At the International Conference on Freshwater in Bonn in 2001, water scarcity was attributed to growing demand and increasing pollution and waste of freshwater sources. There is confusion between water stress at the household and regional scales. Regional water stress is sometimes portrayed as the major determinant of households’ access to adequate water and sanitation, as well as the prevalence of water-related diseases ( UN-Habitat, 2003 ). The amounts of water required to meet basic needs are relatively modest. It is estimated that on a worldwide basis, agriculture accounts for about 69 per cent of annual water withdrawals; industry about 23 per cent, and domestic use about 8 percent ( Hinrichsen et al, 1997 ). In Africa the percentage of domestic water use is estimated to be 7 per cent, while in Asia only 6 per cent ( Hinrichsen et al, 1997 ).
The most common measure for water stress at a national level is the Falkenmark indicator ( Falkenmark et al, 1989 ) which estimates the amount of freshwater available per capita per year. Benchmark values for the Falkenmark indicator are less than 1700m 3 per capita per year, which indicates water stress, while less than 1000m 3 per capita per year indicates severe water stress. In Figure 4-14 the relationship between urban water access, national water stress and national gross domestic product (GDP) per capita is shown based on data from the United Nations Environment Programme (UNEP) Data Compendium ( UNEP, 2002 ). The figure shows the counter-intuitive relationship that water stressed nations have a larger proportion of their populations with access to water than those nations not considered ‘water stressed’. Figure 4-14 shows that it is an erroneous over-simplification to extrapolate freshwater stress at a national level to imply similar reduced access to water at the household level, regardless of the GDP per capita. Apart from a variety of other possible explanations, it is crucial to bear in mind that domestic drinking water supply and sanitation make up a very small fraction of any nation’s demand for water resources.
FIGURE 4-14
Relationship between urban water access, national water stress, and national GDP per capita. SOURCE: UN-Habitat (2003).
Solutions to Boundary Problems?
Boundaries at different levels can be helpful in identifying problems within environmental services. Water provision by a utility to the neighbourhood level, but not to the individual household, invites the creation of an informal sector that may or may not operate as an extortionate cartel. The interface between differing levels can also be critical where the volumes in and out of the interface do not match. In the case of solid waste management, waste may be carried to a neighbourhood bin, but not picked up from that bin by the municipal service. Local drains that serve one neighbourhood by flooding their neighbour can also reflect a failure to consider the whole system. So service provision must extend to the true ‘end user’ in the outer boundaries to ensure that the goals of access are really achieved.
Solutions to boundary problems are rarely obvious, or else sound trite. Nevertheless, two strategies emerge:
- Careful definition of boundaries, with flexible mechanisms for redefining them . Redrawing boundaries along hydrological rather than political lines has worked well in some European watersheds ( Okun, 1978 ). This has resulted in the advocacy for an integral holistic and transboundary management system of river basins ( BHS, 1998 ). Unforeseen problems will, however, still arise, and there must be a reasonable way to refine or redraw the boundaries in the light of experience.
- Good mechanisms to identify and confront cross - boundary problems. Identification of cross-boundary problems is not so difficult, especially if a group is given the task of finding out, for example, why government services don’t perform properly. Resolution of inter-departmental conflicts, however, is a timeless management problem ( Handy, 1985 ). ‘Interdepartmental Task Forces’ can be effective, or simply a sop offered in order to appear to be doing something about the problem.
The problems of the poor, are suffered by the poor, and dealt with by the poor, The problems of the rich, are suffered by the public, and dealt with by the Government. Marianne Kjellen
Scale of the Problem
At present, an estimated 2.6 billion people lack access to improved sanitation and 1.1 billion people lack access to improved water supply. While there is substantial uncertainty about the definition or measurement of these terms, there is no doubt that a very large proportion of the human race does not have what public health authorities could accept as reasonable access to water and sanitation, and thus to the prerequisites for better hygiene. The toll of this inadequate access is high in terms of health, time, money, comfort and dignity. More than 2 million people, mainly children, die every year from diarrhoeal disease, equivalent to a jumbo jet crash every two hours. Unfortunately, this burden falls almost exclusively upon the poor, and most of it could be avoided with promotion of better water, sanitation and hygiene services.
Water, Sanitation, Hygiene and Health
It has been well established for nearly 30 years that the quantity of water that people use for personal and domestic hygiene is more important in maintaining health than the quality of the water they drink. While recent epidemiology on household water treatment justifies greater attention to this intervention, the implications for the public utility remain the same: that physical access to nearby waterpoints is a key aspect of service provision. The importance of excreta disposal and hygiene practices such as handwashing are obvious from the models mentioned and clearly visualized in the F-diagram.
Access to Services
The practical interrelationship between water, sanitation and hygiene in relation to health are far more complex than the theoretical links. This makes health impacts difficult to attribute to improved services, and the measurement of health impacts unsuitable for routine project evaluation. Increasing the access to water and sanitation is widely recognized as leading to health improvements, and new targets have been set by the international community to improve worldwide morbidity and mortality.
So far, the type of technology used at household level has been used as the main proxy for access and health impact. More careful consideration shows that this idea has severe limitations, and that better indicators of access to environmental health services need to be developed. Reasonable access to services such as ‘improved’ water is a complex idea with many facets, encompassing not just water quality, but also its quantity, cost, operational reliability, seasonal availability and collection time and effort. Measuring access to improved services, although essential, is not straightforward. To measure progress on these targets, more powerful and sector specific survey tools will need to be developed, along with the will to use them.
Domestic water consumption increases greatly with convenient and affordable water delivery to the household, reflecting the importance of collection time as a determinant in the amount of water used. These types of proxy indicators are easier to measure than the health impacts obtained by these improved services.
Boundaries, Scales of Environmental Challenges and Access
Before they can be expanded, services have to become more efficient. Institutional boundaries are central to many environmental problems, and are closely linked to the economist’s idea of ‘externalities’, which occur when individuals or agencies are unaffected by the consequences of their actions. In many cases, these boundaries are regarded as immutable, because of institutional resistance to change. Identifying, acknowledging and addressing the various problems associated with institutional boundaries requires time, energy and goodwill. Successful models can evolve only after trial and error, careful monitoring and evaluation and honest documentation of both success and failure.
Environmental health operates on a variety of levels. One simple spatial division is between public and domestic domains of infection. The most vulnerable groups in society in terms of mortality and morbidity (children and the elderly) spend most of their time in the domestic domain. Recent epidemiology of disease transmission and hygiene has stressed the need to create conditions in which households can manage the domestic domain more effectively, through increased water use, household sanitation and improved personal hygiene.
Regional water stress is sometimes portrayed as the major determinant of households’ access to adequate water and sanitation. Household consumption in the world is below 8 per cent of the global water use, and this chapter shows that household access to water in urban areas is, on average, higher in water-stressed nations than in countries where there is no water stress, according to the Falkenmark indicator.
This chapter has also presented a range of scales in which to examine environmental challenges, varying from that of the household up through street, ward and city levels. These scales often reflect their own boundary problems, as when the capacity to collect waste at one point is not equal to the capacity to remove it to the next stage. Householders, public health experts, and infrastructure professionals view these environmental scales differently. Both householders and public health experts see the household as the natural focus for environmental service provision, while infrastructure professionals tend to focus, for good and bad reasons, upon the centralized infrastructure (treatment works, centralized pumping, and so on). Environmental service provision is a chain, and, like all chains, is only as strong as its weakest link. Improvements in environmental health infrastructure will only be significant if they lead to changes at the household level. This public health reality underscores the need for improved access to environmental services at the household level. We need reliable measures of such access if we are to improve it, and the need for better practical indicators of access to environmental services is thus critical for the sector at this time.
The findings, interpretations and conclusions expressed in this article are entirely those of the authors, and should not be attributed to the World Bank.
- MEDICAL RESEARCH AND SOCIAL ENTREPRENEURSHIP COMMUNITIES: INCREASING THE DIALOGUE MAY LEAD TO NEW INSIGHTS FOR PUBLIC HEALTH
Sharon H. Hrynkow, Ph.D. 7
National Institutes of Health
A wave of enthusiasm for “social entrepreneurship” is sweeping across university campuses in the United States, the not-for-profit or citizen sector, and the private sector. While the concepts underpinning social entrepreneurship are not new, having been introduced as a discipline nearly 30 years ago, the vigor with which theses concepts are being applied to tackle tough societal problems is new and increasingly sophisticated. And, the numbers of individuals, nongovernmental organizations (NGOs), and for-profit entities applying the principles are only increasing.
At the same time, the health and medical research community in the United States recognizes that new approaches in the research arena are needed in order to translate basic science knowledge into public health practice. As stated in the 2009 Budget Request Congressional Justification for the National Institutes of Health (NIH), “health care costs will not be tempered unless we accelerate the discovery of transformative ways of practicing medicine—which can only happen through research” ( NIH, 2008 ). The principles and successes of social entrepreneurship, which introduce new and sometimes disruptive ideas and completely new delivery mechanisms, bear examination as nations work to lower health care costs.
This paper examines four strategies to provide clean water or sanitation in resource-poor settings. Each case is examined through the lens of social entrepreneurship and with a view toward identifying lessons learned to inform future efforts in the medical research arena. Examples are not delineated in depth but were chosen to offer illustrative examples. Clean water and sanitation was selected as the topic area in part because of the enormous toll in death, disability, and human suffering exacted due to poor water quality and sanitation: nearly 10 percent of the global burden of disease could be prevented by improving water, sanitation, and hygiene, and in the 32 worst-affected countries, this figure would be 15 percent ( Prüss-Üstün, 2008 ).
What Is a Social Entrepreneur?
Much has been written about social entrepreneurs and the movement in the past two decades, and there have admittedly been many champions. Bill Drayton, founder of Ashoka, the first and one of the best known organizations that invests in social entrepreneurs, states that “There is nothing more powerful than a new idea in the hands of a true social entrepreneur” ( Ashoka, 2009a ). But what is a social entrepreneur? Most agree that social entrepreneurs are “individuals with innovative solutions to society’s most pressing social problems” ( Ashoka, 2009b ). Among the qualities that typify a social entrepreneur, they are determined, ambitious, and persistent; they persuade societies to take new leaps; and they are visionaries and ultimate realists at the same time. In his book How to Change the World: Social Entrepreneurs and the Power of New Ideas , David Bornstein examines the social entrepreneurship wave and offers perspectives on the qualities that typify these innovative leaders: willingness to self-correct, share credit, break free of established structures, cross disciplinary boundaries, work quietly, and with strong ethical impetus ( Bornstein, 2004 ).
Examples of social entrepreneurs are many and they can be found through time. While not necessarily viewed as social entrepreneurs at the time, many well-known historical figures have worked tirelessly to change minds and systems. Florence Nightingale established nursing as a profession, while at the same time transforming how hospitals operate. John Muir fought to preserve and conserve nature’s beauty by changing the way people experienced it, leading to the establishment of the U.S. National Park System and helping to found The Sierra Club. More recently, Nobel Prize winner Muhammad Yunnus showed that lending small amounts of money to impoverished people, against all instincts of traditional banks, can spur sustainable small businesses, lifting families out of hopelessness through enterprise and full economic citizenship, rather than through charity or government services. These visionaries represent only a small sampling of true social entrepreneurs.
Many foundations have realized the role of social entrepreneurs as mass mobilizers of citizens and as good value for the initial investment, leveraging dollars with the promise of high impact. There are now more than a dozen major grant-making organizations devoted specifically to the support of social entrepreneurs, in addition to Ashoka’s focus on institution building and transformation of the entire citizen sector in entrepreneurial directions. Efforts to provide sustainable energy and clean water in remote villages, to conserve rainforests, to ensure quality education for all, to dismantle landmines, and to teach children living in poverty how to become self-sufficient are just some of the challenges being supported by social entrepreneur organizations. Efforts in the not-for-profit sector are complemented by those in the private sector, which has also taken up the mantle of social entrepreneurship. Bill Gates, Jr., is in every sense a business entrepreneur, but through the Bill and Melinda Gates Foundation he is working for the global public good through a range of innovative, large-scale programs. Furthermore, the for-profit sector has increasingly incorporated concepts of social entrepreneurship into corporate strategies: FastCompany and others track the social entrepreneurial movement embedded within the private sector. 8 Between 1990 and 2005, FastCompany trends show that for-profit companies involved in social entrepreneurship grew from about a dozen to more than 30. And more sophisticated approaches toward social entrepreneurship are being developed and tested. For example, Ashoka is now developing new approaches for combining multiple social entrepreneurs and innovative ideas in creative forums in order to refine and accelerate the most promising ideas and to spark entirely new insights based on the dynamic engagement of multiple entrepreneurs working together.
Clean Water and Sanitation—Consideration of Two Social Entrepreneurial Approaches
Boxes 4-2 and 4-3 provide snapshots of two Ashoka Fellows in Indonesia, each of whom approached the clean water challenge differently. 9 Both cases represent operational strategies related to collection and use of water data or to provision and marketing of sanitation services. In one case, river water was collected and analyzed from multiple locations across the span of several years to show the burden of pollution to nearby inhabitants. The volume of credible data collected by ordinary citizens, including school children, in a methodical and persistent way was used as a platform to engage journalists and policy makers, leading to reform. The second example focuses on delivery of sanitation measures in an urban slum. Using a business model and training for a local family, the program led to sustained used of toilet facilities for a small fee. Anecdotal evidence suggests that health was improved due to reductions of raw sewage in the streets.
Communities Develop Evidence Base for Water Quality Policies. Ashoka Fellow Prigi Arasandi works in 51 Indonesian villages with the goal of cleaning the water of the Surabaya River, a large drainage from the interior (more...)
Community Leadership to Improve Sanitation. Ashoka Fellow Hamzah Harum Al’Rasyid works in poor urban areas in Indonesia to provide “community-based sanitation centers.” Mr. Al’Rasyid works with (more...)
There are many strengths in the social entrepreneur approach as currently deployed in both cases in Indonesia. First, both models depend on a firm understanding of local communities, cultures, and norms. Ashoka Fellows, who themselves are from the same countries in which they conduct their work, spend time with community members to define project parameters, gain trust, and learn essential insights for successful implementation. Second, both models turn on its head the expectation of whose job it is to solve social problems, engaging community members as “experts,” not just for the purpose of consultation but to invest community members with decision-making authority. Project development is informed by the community experts and then executed in close partnership with them. Both programs valued community members from the start, to be consulted and respected and vested with responsibility instead of treating them as uninformed future beneficiaries. At least in part because of this approach, important cobenefits were realized: The citizen base developed feelings of ownership for the river and its quality in the first case, which led to further direct river stewardship actions and to mobilization as a political constituency when needed. In the second case, cobenefits included awareness of and pride in, and sometimes substantial revenue from, the health benefits of good sanitation measures. Mr. Al’Rasyid’s project, along with a handful of other social entrepreneurs in other countries, 10 also shows that very poor people are willing to pay to use community toilets. Because of the delivery methodology used, locals report feelings of ownership and interest in helping to keep the facilities clean and well maintained.
Third, solid data is the foundation of both programs. In Mr. Arisandi’s work, schools along the river were identified and teachers were trained to teach children about hydrology, water use and sources, and environmental connectedness through classroom work and field trips. This was complemented by additional outreach to those not associated with schools. Indeed, one of the foundations to the success of the whole effort was in convincing citizens, often uneducated adults or children, to rigorously test water quality at more than 50 sites for several years and in a way which is credible (using bioindicators). With large volumes of credible data, efforts to engage policy makers were facilitated. And, not incidentally, the process of collecting those data from the citizen base also created an important political constituency that then became influential in the public policy process.
Fourth, in the case of the river clean-up, the engagement of journalists to help spread information and awareness about the effort was an integral and successful component. Mr. Arisandi provided weekly information updates to 14 journalists who then collaborated with him on pieces for a range of outlets. Such outreach paved the way for policy maker involvement, leading directly to new laws requiring the monitoring of the river’s water quality.
Finally, both cases point to the benefits of systems thinking. What changed in each case was not so much the data collected or the service provided but rather the ways in which the business was conducted. This comprehensive approach places the effort on a broader backdrop of activity, thereby integrating it more fully into the fabric of the community. It successfully puts the financial, educational, and other incentives directly in front of the people whose behavior needs to change in order to achieve the goals. Furthermore, once the work is understood in this way, both systemic models can be replicated with appropriate modification in other locales.
Rigorous evaluation of the programs has not yet been completed. While anecdotal evidence points to success, evaluation may provide insights that could further strengthen the programs and suggest future actions.
Clean Water—Consideration of Two Health Research Approaches
Many of the qualities ascribed to social entrepreneurs may be attributed to those health researchers who have worked over long periods to achieve success on a single goal. As social entrepreneurs, medical researchers can best be described as “driven,” “willing to work across confines of fields and geography,” and having “long-term vision,” as just some examples of the similarities. The work of two such “driven” researchers is outlined in Boxes 4-4 and 4-5 . Dr. Rita Colwell has contributed to the knowledge base to understand the life cycle of the pathogenic agent for cholera, Vibrio cholerae , and its copepod vector. Over decades, she and her colleagues showed that a variety of characteristics of water, including temperature and salinity, affect the spread of the cholera bacterium. She also showed that the copepod vector could be effectively filtered out using readily available sari cloth in poor villages, leading to reduced illness.
A Multidisciplinary Approach to Prevent Cholera. Dr. Rita Colwell, now at the University of Maryland, and her colleagues have studied transmission dynamics of cholera in the Bangladeshi villages for more (more...)
Basic and Behavioral Science to Reduce Arsenic Exposures. Dr. Joseph Graziano and his colleagues have worked over many years to understand the dose-response relationships between exposure to arsenic and (more...)
Dr. Joseph Graziano and his colleagues provided critical insights into the health impacts of arsenic, including the molecular mechanisms underlying disease. Recent work ( Gamble et al., 2006 ) has led to interventional studies focused on folic acid as a means to assist subsets of the population in metabolizing arsenic to inactive forms. Moving from the bench back to the field, Dr. Graziano and his colleague Dr. Alexander van Green worked with a community-based effort to localize wells with high- or low-arsenic levels. With thousands of participants and attendant data, it was found that within a single village both high- and low-arsenic wells can exist. More recent studies have shown that, even when presented with information on arsenic impacts, other factors come into play when determining whether to use water from high- or low-arsenic wells. While roughly half of residents with high-arsenic wells switched to low-arsenic wells, others did not, largely because the distances to the safer wells were too long. The “durability” of well-switching over time is not clear, and other options such as the provision of a deep, low-arsenic community well appear to have much greater promise as a longer term solution.
Both of these examples show the impact and import of sustained hypothesis-driven research efforts over time. With the dedicated support and vision of these medical researchers over decades, new knowledge was gained to underpin intervention efforts related to cholera and arsenic-related disease. Both examples also show the ability of the researchers to engage communities as part of the interventional research. In the case of the folded sari intervention for cholera, the community played a leadership role in ensuring that the interventional pilot study was conducted. Some in the research community were concerned that the pilot would fail since it was believed that men would not drink water that had been filtered through sari cloth. It was discovered, however, that men had already been using the same sari cloth as part of the process for their local production of fermented drinks. In this case, the community voice allowed an intervention to be tested that might otherwise have been left off the drawing board.
In terms of scale-up and long-term adoption of the interventions, both examples illustrate the challenges in moving from a basic research understanding of a problem to a populations-based intervention strategy. In the case of the folded sari intervention, despite its clear effectiveness in filtering out the cholera vector, and demonstrated reduction in illness after filtering, uptake of the intervention was sustainable in that filtration continued after the project was completed, but the details, namely, the number of folds effective for filtering out the plankton were not adhered to in every case. Similarly, there was a lack of permanent switching to the use of low-arsenic wells. Two challenges present themselves. First, the incomplete uptake of the intervention may be due to a lack of local reinforcement of the links between clean water and better health within the community. Continued efforts to raise awareness are needed. Second, given the number of donors working in the region, questions may also be raised as to why the simple sari intervention or well-switching interventions were not incorporated into larger development programs. Placing these simple interventions into broader development perspective bears examination.
The global burden of illness and death directly related to lack of clean water and sanitation demands that we consider all possible strategies. As various groups take on this challenge, new ideas and programs will be put in place. By merging the best features of different approaches, even better strategies may surface.
This paper juxtaposes two approaches to improving clean water and sanitation in resource-poor settings. The social entrepreneur approach focuses on delivery of interventions or gathering of information for policy purposes. The medical research approach focuses on understanding the links between toxins or microbes and attendant ill health, then working to mitigate exposures. Both approaches have yielded immense data sets and new knowledge that has led to improved health.
There are similarities and differences between the approaches. Some of the key features of the two approaches are outlined in Table 4-9 . Closer examination of two features in particular provide insights into potential future actions. First, how community members were included in the work had an impact on the overall outcomes. The social entrepreneurship model embraces community perspectives as true experts, thereby ensuring that voices heard from the earliest of stages are those from ultimate beneficiaries, and that the work witnesses and subsequently accounts for the incentives faced by those whose social behavior it seeks to change. Interventions based on community involvement had a high degree of uptake. This particular approach leads to feelings of “ownership” of the work, driven by educational or economic incentives, and efforts to ensure its sustainability. The medical research model includes important elements of community engagement, particularly during data gathering for pilot efforts and as part of educational efforts related to scale-up of interventions. Long-term sustainability of effective interventions might improve if community engagement were viewed through another lens. Increased community involvement, including consults with local social entrepreneurs, may lead to more effective and long-lasting uptake of interventions, both geographically and on a long-term timescale. One can easily imagine the impact that the folded sari intervention might have in the hands of a social entrepreneur. By the same token, linking information about low- and high-arsenic wells in particular villages to motivated community members or social entrepreneurs might yield new insights on critical operational aspects.
Key Features of the Two Approaches on Clean Water and Sanitation.
Second, the type of funding for the two approaches dictates in many ways the range of activities that may be supported. Medical research grants supported by governments tend to limit activities to those that lead to new knowledge about population-based health risks, outcomes due to exposures, including cellular and subcellular responses, and effectiveness of clinical interventions, for example. The development of policy-relevant data has not been a traditional focus of medical research grants. Given the priorities, funding cycles tend to be on the four- to five-year range. The researchers described in the present text pieced together long-term funding strategies over time using multiple sources. This is in contrast to the Ashoka model, which provides support with relatively few conditions for a critical phase of start-up activity intended to lead to population-based policy or intervention results and intended to get the entrepreneurial work to a solid enough footing that more traditional organizations will step in to support and/or replicate. Looking at the two models, it could be speculated that some medical researchers would benefit from a granting system that provided longer term support, perhaps on a 10-year cycle, which included a mid-term review timed to moving basic science knowledge into practice. Such an approach could capture the best elements of both systems.
Increasing the dialogue between the medical research community and the social entrepreneur community would likely enhance operations on both sides. Formal and informal means could be identified for exchange of views and expertise. Such exchanges might lead to exciting new actions and programs. Possible mechanisms to bolster communication are many and include, first, nominations of social entrepreneurs to public slots on governmental research agency advisory boards and, second, linking social entrepreneurs on the ground with researchers funded through medical research councils or other national or international grant-making bodies. In the latter case, ambassadors, aid mission directors, and other senior government officials, including military, agricultural, and health attachés, could play a role in brokering and facilitating the exchange of ideas between the two communities on the ground. Third, efforts to raise awareness within the medical research community about the social entrepreneurship movement through conferences and lectures would spark ideas for action, particularly from those already attuned to this wave—the next generation. Finally, medical researchers, public health professionals, students, and others should be made aware of new tools such as Changemakers.com, a partnership supported by Ashoka, the Robert Wood Johnson Foundation, and the Global Water Challenge. Through that tool, ideas and entrepreneurial approaches to providing clean water and other seemingly intractable problems, including strengthening of health-care systems, have been identified. Encouraging deposits of good ideas and mining the site for good ideas would be useful undertakings. This would in fact be in line with the next-generation vision of Ashoka, “Everyone is a Changemaker.”
- Acknowledgments
I am very grateful to Rita Colwell and Joseph Graziano for allowing me to tell their stories in this context, and also for their generosity in reviewing and editing the draft paper. David Strelneck of Ashoka provided invaluable insights at every step of the way. He and former Ashoka colleague Carol Grodzins reviewed the draft and made extremely helpful suggestions. Appreciation also goes to NIH colleagues Patricia Mabry (OBSSR) and Claudia Thompson (NIEHS) for their guidance and perspectives early on in the framing of this effort.
- OVERVIEW REFERENCES
- Clasen TF. Scaling up household water treatment: looking back, seeing forward. Geneva: WHO; 2008.
- Clasen TF, Schmidt WP, Rabie T, Roberts I, Cairncross S. Interventions to improve water quality for preventing diarrhoea: systematic review and meta-analysis. British Medical Journal. 2007a; 334 (7597):782. [ PMC free article : PMC1851994 ] [ PubMed : 17353208 ]
- Clasen TF, Haller L, Walker D, Bartram J, Cairncross S. Cost-effectiveness of water quality interventions for preventing diarrhoeal disease in developing countries. Journal of Water and Health. 2007b; 5 (4):599–608. [ PubMed : 17878570 ]
- Clasen T, McLaughlin C, Nayaar N, Boisson S, Gupta R, Desai D, Shah N. Microbiological effectiveness and cost of disinfecting water by boiling in semi-urban India. American Journal of Tropical Medicine and Hygiene. 2008; 79 (3):407–413. [ PubMed : 18784234 ]
- Marcotullio PJ, McGranahan G, editors. Scaling urban environmental challenges: from local to global and back. London: Earthscan Publications; 2007.
- WHO (World Health Organization). International network to promote household water treatment and safe storage. Geneva: WHO; 2008. [ PMC free article : PMC3323158 ] [ PubMed : 15224679 ]
- SINGER REFERENCES
- AWWA (American Water Works Association). Water chlorination/chloramination practices and principles. M20. Denver, CO: AWWA; 2006.
- Boyer TH, Singer PC. A pilot-scale evaluation of magnetic ion exchange treatment for removal of natural organic material and inorganic anions. Water Research. 2006; 40 (15):2865–2876. [ PubMed : 16844182 ]
- Letterman R, editor. Water quality and treatment. 5th edition. Denver, Co: AWWA; 1999.
- MWH. Water treatment principles and design. 2nd edition. Hoboken, NJ: John Wiley & Sons; 2005.
- CARAVATI ET AL. REFERENCES
- ABB Ltd. A2Z of H2O guide to water cycle. 2007. [accessed February 17, 2009]. http://www .tagteam.com /tagteam/client/detail .asp?dbid=539&siteid =836048&dataid =118594 .
- AWWA (American Water Works Association). Water quality and treatment: a handbook of community public water supplies. 5th ed. New York: McGraw-Hill; 1999.
- Bayliss K, McKinley T.Privatising basic utilities in sub-Saharan Africa: the MDG impact. United Nations Development Programme and International Policy Center Policy Research Brief No. 3. 2007. [accessed February 17, 2009]. http://www .undp-povertycentre .org/pub/IPCPolicyResearchBrief003.pdf .
- Black M.Sanitation: creating a stink about the world’s wastewater. The Guardian. 2008. [accessed February 17, 2009]. http://www .guardian.co .uk/environment/2008/aug/20/water .waste .
- Cardone R, Fonseca C.Experiences with innovative financing: small town water supply and sanitation service delivery. Background Paper prepared for UN-HABITAT publication, Meeting Development Goals in Small Urban Centres: Water and Sanitation in the World’s Cities 2006. 2006. [accessed February 17, 2009]. http://www .irc.nl/content /download/26959 /289739/file/UN_Habitat _Innovative_Finan.pdf .
- Crites R, Tchobanoglous G. Small and decentralized wastewater management systems. New York: McGraw-Hill; 1998.
- EPA (Environmental Protection Agency). New York City watershed partnership. 2006. [accessed February 17, 2009]. http://www .epa.gov/NCEI /collaboration/nyc.pdf .
- EPA (Environmental Protection Agency). Benefits of water efficiency. 2009. [accessed February 17, 2009]. http://www .epa.gov/watersense /water/benefits.htm .
- George R. The big necessity: the unmentionable world of human waste and why it matters. New York: Metropolitan Books; 2008.
- Gleick PH. The changing water paradigm: a look at twenty-first century water resources development. Water International. 2000; 25 (1):127–138.
- Gleick PH. The world’s water 2006–2007: the biennial report on fresh water resources. Washington, DC: Island Press; 2006.
- Hightower M, Pierce SA. The energy challenge. Nature. 2008; 452 (7185):285–286. [ PubMed : 18354460 ]
- Hillie T, Munasinghe M, Hope M, Deraniyagala Y. Nanotechnology, water and development. 2006. [accessed February 17, 2009]. http://www .merid.org /nano/waterpaper/NanoWaterPaperFinal.pdf .
- Hutton G, Haller L, Bertram J.Economic and health effects of increasing coverage of low cost water and sanitation interventions. Human Development Report Office Occasional Paper. 2006. [accessed February 17, 2009]. http://hdr .undp.org/en /reports/global/hdr2006/papers/who.pdf .
- IEA (International Energy Agency). World energy outlook 2008. Paris: IEA; 2008.
- Morgan JP.Consultative Group to Assist the Poor. Microfinance: shedding light on microfinance equity valuation: past and present. 2009. [accessed February 17, 2009]. http://www .jpmorgan.com /pages/jpmorgan/investbk/research/mfi .
- Lantagne DS, Quick R, Mintz E. Household water treatment andsafe storage options in developing countries: a review of current implementation practices. Wilson Center Environmental Change and Security Program; 2006. [accessed February 17, 2009]. http://www .irc.nl/page/37316 .
- Logan BE, Regan JM. Microbial fuel cells: challenges and applications. Environmental Science and Technology. 2006; 40 (17):5172–5180. [ PubMed : 16999086 ]
- Lovley DR. Microbial fuel cells: novel microbial physiologies and engineering approaches. Current Opinion in Biotechnology. 2006; 17 (3):327–332. [ PubMed : 16679010 ]
- McKinsey Global Institute. Curbing global energy demand growth: the energy productivity opportunity. 2007. [accessed February 17, 2009]. http://www .mckinsey.com /mgi/publications /Curbing_Global_Energy/index.asp .
- Morgan Stanley. Blue orchard loans for development 2007-1 (“BOLD 2”) prices. 2007. [accessed February 17, 2009]. http://www .morganstanley .com/about/press/articles/4977.html .
- NWP (Netherlands Water Partnership). Microfinance for water, sanitation and hygiene. 2007. [accessed February 17, 2009]. http://www .irc.nl/content /download/128991 /353215/file/Microfinance_%20India.pdf .
- OECD (Organisation for Economic Co-operation and Development). OECD Environmental Outlook to 2030. 2008. [accessed February 17, 2009]. http://www .oecd.org/dataoecd /29/33/40200582.pdf .
- Platz D, Schroeder F. Dialogue on Globalization Occasional Papers 34. New York: Friedrich-Ebert-Stiftung; 2007. Moving beyond the privatization debate.
- Revenga C. The next big ideas in conservation: paying water’s real costs. 2009. [accessed February 17, 2009]. http://www .nature.org /tncscience/bigideas/people/art23907 .html .
- Revenga C, Murray S, Abramowitz J, Hammond A. Watersheds of the world: ecological value and vulnerability. Washington, DC: World Resources Institute; 1998.
- Schnoor J. Living with a changing water environment. The Bridge. 2008; 38 (3):46–54.
- Shannon MA, Bohn PW, Elimelech M, Georgiadis JG, Marinas BJ, Mayes AM. Science and technology for water purification in the coming decades. Nature. 2008; 452 (7185):301–310. [ PubMed : 18354474 ]
- Tchobanoglous G, Burton FL, Stensel HD. Wastewater engineering: treatment and reuse. 4th edition. New York: McGraw-Hill; 2003.
- USGS (United States Geological Survey). Estimated use of water in the United States in 2000. 2005. [accessed February 17, 2009]. http://pubs .usgs.gov /fs/2005/3051/pdf/fs2005-3051.pdf .
- USGS (United States Geological Survey). The water cycle. 2008. [accessed February 17, 2009]. http://ga .water.usgs .gov/edu/watercycle.html .
- Viessman W, Hammer MJ. Water supply and pollution control. 4th edition. New York: Harper and Row; 1985.
- WHO (World Health Organization). Access to improved drinking-water sources and to improved sanitation (percentage). 2008. [accessed February 18, 2009]. http://www .who.int/whosis /indicators/compendium /2008/2wst/en/
- World Bank. Private participation in infrastructure database, 2008. [accessed February 17, 2009]. http://ppi .worldbank.org/
- World Water Assessment Programme. Water for people, water for life. New York: Berghahn Books; Paris: UNESCO; 2003.
- World Water Assessment Programme. Water, a shared responsibility. New York: Berghahn Books; Paris: UNESCO; 2006.
- Zimmerman JB, Mihelcic JR, Smith J. Global stressors on water quality and quantity. Environmental Science and Technology. 2008; 42 (12):4247–4254. [ PubMed : 18605540 ]
- BOSTOEN ET AL. REFERENCES
- Bern C, Martines J, Zoysa ID, Glass RI. ‘The magnitude of the global problem of diarrhoeal disease: A 10-year update’ Bulletin of WHO. 1992; 70 (6):705–714. [ PMC free article : PMC2393403 ] [ PubMed : 1486666 ]
- BHS. University of Exeter, British Hydrological Society; 1998. ‘International Conference on Hydrology in a Changing Environment’ www .hydrology.org.uk/exeter.html .
- Briscoe J, Feachem RG, Rahman M. Measuring the Impact of Water Supply and Sanitation Facilities on Diarrhoea Morbidity; Prospects for Case-control Methods. World Health Organization (WHO), Environmental Health Division; Geneva: 1985.
- Cairncross S. ‘Water supply and sanitation: An agenda for research’ Journal of Tropical Medicine and Hygiene. 1989; 92 :301–314. [ PubMed : 2681813 ]
- Cairncross S. ‘Health impacts in developing countries: New evidence and new prospects’ Journal of the Institution of Water and Environmental Management. 1990; 4 (6):571–577.
- Cairncross S. Safe Water Environments. Swedish International Development Cooperation Agency (Sida); Eldoret: 1995. Water Quality, Quantity and Health.
- Cairncross S. Measuring the Health Impact of Water and Sanitation. London: WEDC, London School of Hygiene and Tropical Medicine (LSHTM); 1999. p. 2. www.worldbank.org/watsan/pdf/tn02.pdf, www.lboro.ac.uk/well/resources/fact-sheets/fact-sheets-htm/mthiws.htm .
- Cairncross S, Blumenthal U, Kolsky P, Moraes L, Tayeh A. ‘The public and domestic domains in the transmission of disease’ Tropical Medicine and International Health. 1996; 1 (1):27–34. [ PubMed : 8673819 ]
- Cairncross S, Feachem RG. Environmental Health Engineering in the Tropics. John Wiley & Sons; Chichester: 1993.
- Clasen T. PhD thesis. LSHTM; London: 2006. ‘Household water treatment for the prevention of diarrhoeal disease. Department of Infectious Diseases’ p. 271.
- Clasen TF, Bastable A. ‘Faecal contamination of drinking water during collection and household storage: The need to extend protection to the point of use’ Journal of Water and Health. 2003; 1 (3):109–115. [ PubMed : 15384721 ]
- Clasen TF, Cairncross S. ‘Editorial: Household water management: Refine the dominant paradigm’ Tropical Medicine and International Health. 2004; 9 (2):187–191. [ PubMed : 15040554 ]
- Clasen T, Roberts I, Rabie T, Cairncross S. Interventions to Improve Water Quality for Preventing Infectious Diarrhoea. Cochrane Library, Infectious Diseases Group, John Wiley & Sons; Chichester: 2004.
- Conroy RM, Meegan ME, Joyce T, McGuigan K, Barnes J. ‘Solar disinfection of drinking water protects against cholera in children under 6 years of age’ Archives for Diseases in Childhood. 2001; 85 (4):293–295. [ PMC free article : PMC1718943 ] [ PubMed : 11567937 ]
- Curtis V, Cairncross S, Yonli R. ‘Domestic hygiene and diarrhoea – pinpointing the problem’ Tropical Medicine and International Health. 2000a; 5 (1):22–23. [ PubMed : 10672202 ]
- Curtis V, Cairncross S, Yonli R. ‘Domestic hygiene and diarrhoea – pinpointing the problem’ Tropical Medicine and International Health. 2000b; 5 (1):22–32. [ PubMed : 10672202 ]
- Deb BC, Sircar BK, Sengupta PG, De SP, Mondal SK, Gupta DN, Saha NC, Ghosh S, Mitra U, Pal SC. ‘Studies on interventions to prevent el tor cholera transmission in urban slums’ Bulletin of the World Health Organization. 1986; 64 (1):127–131. [ PMC free article : PMC2490926 ] [ PubMed : 3488134 ]
- Esrey SA, Feachem RG, Hughes JM. ‘Interventions for the control of diarrhoeal diseases among young children: Improving water supplies and excreta disposal facilities’ Bulletin of the World Health Organization. 1985; 63 (4):757–772. [ PMC free article : PMC2536385 ] [ PubMed : 3878742 ]
- Esrey SA, Potash JB, Roberts L, Shiff C. ‘Effects of improved water supply and sanitation on ascariasis, diarrhoea, dracunculiasis, hookworm infection, schistosomiasis and trachoma’ Bulletin of the World Health Organization. 1991; 69 (5):609–621. [ PMC free article : PMC2393264 ] [ PubMed : 1835675 ]
- Falkenmark M, Lundqvist J, Widstrand C. ‘Macro-scale water scarcity requires micro-scale approaches: Aspects of vulnerability in semi-arid development’ Natural Resources Forum. 1989; 13 (4):258–267. [ PubMed : 12317608 ]
- Feachem RG, Bradley DJ, Garelick H, Mara DD. Sanitation and Disease; Health Aspects of Excreta and Wastewater Management. John Wiley & Sons; Chichester: 1983a.
- Feachem RG, McGarry M, Mara D, editors. Water, Waste and Health in Hot Climates. John Wiley & Sons; Chichester: 1977.
- Feachem RG, Hogan RC, Merson MH. ‘Diarrhoeal disease control: Reviews of potential interventions’ Bulletin of the World Health Organization. 1983b; 61 (4):637–640. [ PMC free article : PMC2536145 ] [ PubMed : 6354505 ]
- Fewtrell L, Kaufmann RB, Kay D, Enanoria W, Haller L, Colford JM Jr. ‘Water, sanitation, and hygiene interventions to reduce diarrhoea in less developed countries: A systematic review and meta-analysis’ Lancet Infectious Diseases. 2005; 5 (1):42–52. [ PubMed : 15620560 ]
- Handy CB. Understanding Organizations. Penguin Books; London: 1985.
- Hardin G. ‘The tragedy of the commons’ Science. 1968; 162 :1243–1248. [ PubMed : 5699198 ]
- Hellard ME, Sinclair MI, Forbes AB, Fairley CK. ‘A randomized, blinded, controlled trial investigating the gastrointestinal health effects of drinking water quality’ Environmental Health Perspectives. 2001; 109 (8):773–778. [ PMC free article : PMC1240403 ] [ PubMed : 11564611 ]
- Hinrichsen D, Robey B, Upadhyay UD. Solution for a Water-Short World. Baltimore, MA: Johns Hopkins School of Public Health, Population Program; 1997. www .infoforhealth.org/pr/m14edsum.shtml .
- Iijima Y, Karama M, Oundo JO, Honda T. ‘Prevention of bacterial diarrhea by pasteurization of drinking water in Kenya’ Microbiology and Immunology. 2001; 45 (6):413–416. [ PubMed : 11497215 ]
- Jagals P, Grabow WOK, Williams E. ‘The effects of supplied water quality on human health in an urban development with limited basic subsistence facilities’ Water South Africa. 1997; 23 (4):373–378.
- Jonnalagadda PR, Bhat RV. ‘Parasitic contamination of stored water used for drinking/cooking in Hyderbad’ South-East Asian Journal of Tropical Medicine and Public Health. 1995; 26 (4):789–794. [ PubMed : 9139396 ]
- Kirchhoff LV, McClelland KE, Do Carmo Pinho M, Araujo JG, De Sousa MA, Guerrant RL. ‘Feasibility and efficacy of in-home water chlorination in rural Northeastern Brazil’ Journal of Hygiene. 1985; 94 (2):173–180. [ PMC free article : PMC2129411 ] [ PubMed : 2985691 ]
- Kolsky PJ. ‘Diarrhoeal disease: Current concepts and future challenges. Water’ Transactions of the Royal Society of Tropical Medicine and Hygiene. 1993; 87 (supplement no 3):43–46. [ PubMed : 8108850 ]
- Kolsky P.‘Water, health and cities: Concepts and examples’. Paper presented at an international Workshop on Planning for Sustainable Development: Cities and Natural Resource Systems in Developing Countries; 13–17 July, 1992; Cardiff: University of Wales; 2002.
- Lewin S, Stephens C, Cairncross S. Review prepared for the Overseas Development Administration. LSHTM; London: 1996. ‘Health impacts of environmental improvements in Cuttack and Cochin, India’
- McGranahan G, Jacobi P, Songsore J, Surjadi C, Kjellen M, editors. The Citizens at Risk: From Urban Sanitation to Sustainable Cities. Earthscan; London: 2001.
- Mintz ED, Reiff FM, Tauxe RV. ‘Safe water treatment and storage in the home. A practical new strategy to prevent waterborne disease’ Journal of the American Medical Association. 1995; 273 (12):948–953. [ PubMed : 7884954 ]
- Murray CJL, Lopez AD, editors. The Global Burden of Disease: A Comprehensive Assessment of Mortality and Disability from Diseases, Injuries, and Risk Factors in 1990 and Projected to 2020. Harvard University Press; Harvard, MA: 1996.
- Okun DA. The Regionalization of Water Management: A Revolution in England and Wales. Applied Science Publishers; London: 1978.
- Quick RE, Venczel LV, Mintz ED, Soleto L, Aparicio J, Gironaz M, Hutwagnar L, Greene K, Bopp C, Maloney K, Chavez D, Sobsey M, Tauxe R. ‘Diarrhoea prevention in Bolivia through point of use water treatment and safe storage: A promising new strategy’ Epidemiology and Infection. 1999; 122 :83–90. [ PMC free article : PMC2809591 ] [ PubMed : 10098789 ]
- Ruff LE. ‘The economic common sense of pollution’ The Public Interest. 1970 Spring; 19 :69–85.
- Thompson J, Porras IT, Tumwine JK, Mujwahuzi MR, Katui-Katua M, Johnstone N, Wood L. Drawers of Water: 30 Y ears of Change in Domestic Water Use and Environmental Health – Summary, Earthprint. 2002. www .iied.org/sarl/pubs/drofwater .html#9049IIED?to=9049IIED ; www .iied.org/sarl/dow .
- UNEP (United Nations Environment Programme). Global Environmental Outlook 3. Earthscan; London: 2002.
- UN-HABITAT (United Nations Human Settlements Programme). Water and Sanitation in the World’s Cities. Earthscan; London: 2003.
- VanDerslice J, Briscoe J. ‘All coliforms are not created equal: A comparison of the effects of water source and in-house water contamination on infantile diarrhoeal disease’ Water Resources Research. 1993; 29 (7):1983–1993.
- Wagner EG, Lanoix JN. WHO monograph series. Geneva: 1958. ‘Excreta disposal for rural areas and small communities’ p. 39. [ PubMed : 13581743 ]
- White GF, Bradley DJ, White AU. Drawers of Water. University of Chicago Press; Chicago, IL: 1972.
- WHO. Minimum Evaluation Procedure (MEP) for Water Sanitation Projects. World Health Organization; Geneva: 1983. p. 52.
- WHO. The World Health Report 2001; Mental Health: New Understanding, New Hope. Geneva: World Health Organization; 2001. www .who.int/whr/
- WHO. The World Health Report 2004 – Changing History. Geneva: WHO; 2004. www .who.int/whr/2004/en/index.html .
- WHO/UNICEF (United Nations Children’s Fund). Global Water Supply and Sanitation Assessment 2000 Report. 2000. www .unicef.org/programme /wes/pubs/global/global.htm .
- WHO/UNICEF. Making it Happen. Geneva: World Health Organization; 2005. Water for Life. www .who.int/water_sanitation_health /waterforlife.pdf .
- WHO/UNICEF. Meeting the MDG Drinking Water and Sanitation Target: The Urban and Rural Challenge of the Decade. 2006. www .childinfo.org/areas /water/pdfs/jmp06final.pdf .
- World Bank. Measurement of the Health Benefits of Investments in Water Supply. The World Bank; Washington, DC: 1976.
- HRYNKOW REFERENCES
- Ashoka. Bill Drayton: nothing more powerful. 2009a. [accessed February 12, 2009]. http://www .ashoka.org/video/4108 .
- Ashoka. What is a social entrepreneur? 2009b [accessed February 12, 2009]; http://www .ashoka.org /social_entrepreneur .
- Bornstein D. How to change the world: social entrepreneurship and the power of new ideas. New York: Oxford Univesity Press; 2004.
- Colwell RC, Hug A, Islam MS, Aziz KM, Yunus M, Khan NH, Mahmud A, Sack RB, Nair GB, Chakraborty J, Sack DA, Russek-Cohen E. Reduction of cholera in Bangladeshi villages by simple filtration. Proceedings of the National Academy of Sciences. 2003; 100 (3):1051–1055. [ PMC free article : PMC298724 ] [ PubMed : 12529505 ]
- Gamble MV, Liu X, Ahsan H, Pilsner JR, Ilievski V, Slavkovich V, Parvez F, Chen Y, Levy D, Factor-Litvak P, Graziano JH. Folate and arsenic metabolism: a double-blinded, placebo-controlled folic acid-supplementation trial in Bangladesh. American Journal of Clinical Nutrition. 2006; 84 (5):1093–1101. [ PMC free article : PMC2046214 ] [ PubMed : 17093162 ]
- Khan AW, Akhtar SK, Ahmad MH, Sayed SU, Hadi SKA, Khan MH, Jalil MA, Ahmed R, Faruquee MH. Arsenic contamination in groundwater and its effect on human health with particular reference to Bangladesh. Journal of Preventive and Social Medicine. 1997; 16 (1):65–73.
- Mead MN. Columbia programs digs deeper into arsenic dilemma. Environmental Health Perspectives. 2005; 113 (6):A374–377. [ PMC free article : PMC1257618 ] [ PubMed : 15929878 ]
- NIH (National Institutes of Health). Summary of the FY 2009 President’s Budget. 2008. [accessed February 12, 2009]. http: //officeofbudget .od.nih.gov/ui/2008 /Summary%20of%20FY%202009 %20Budget-Press%20Release.pdf .
- Prüss-Üstün A, Bos R, Gore F, Bartram J. Safer water, better health: costs, benefits and sustainability of interventions to protect and promote health. Geneva: World Health Organization; 2008.
- WHO (World Health Organization). Weekly epidemiological record. 2006. [accessed April 6, 2009]. www .who.int/wer/2006/wer8131.pdf .
- WHO (World Health Organization). Cholera in Zimbabwe—update. 2008. [accessed April 6, 2009]. http://www .who.int/csr /don/2008_12_26/en/index.html .
Dan Okun Distinguished Professor of Environmental Engineering, Department of Environmental Sciences and Engineering, Gillings School of Global Public Health.
CT = product of free chlorine residual (C) and contact time (T) required for disinfection.
School of Civil and Environmental Engineering.
Schools of Civil and Environmental Engineering, and Material Science and Engineering. Corresponding author may be contacted at Georgia Institute of Technology School of Civil and Environmental Engineering, 790 Atlantic Dr. N.W., Atlanta, GA 30332-0355, Phone: (404) 894-2201, Fax: (404) 894-2278, E-mail: [email protected] .
The conversion of liquid to gas.
This paper is reprinted with permission from Bostoen, K., P. Kolsky, and C. Hunt. 2007. Improving urban water and sanitation services—health, access, and boundaries. In Scaling urban environmental challenges: from local to global and back , edited by P. J. Marcotullio and G. McGranahan. London: Earthscan Publications.
Associate director, National Institute of Environmental Health Sciences.
See http://www .fastcompany.org .
For additional details on each Fellow’s work, see www .ashoka.org .
See Ashoka Fellows David Kuria in Kenjya and Isaac Durojaiye in Nigeria, www .ashoka.org .
- Cite this Page Institute of Medicine (US) Forum on Microbial Threats. Global Issues in Water, Sanitation, and Health: Workshop Summary. Washington (DC): National Academies Press (US); 2009. 4, Addressing Risk for Waterborne Disease.
- PDF version of this title (13M)
In this Page
- IMPROVING URBAN WATER AND SANITATION SERVICES: HEALTH, ACCESS, AND BOUNDARIES
Other titles in this collection
- The National Academies Collection: Reports funded by National Institutes of Health
Related information
- PMC PubMed Central citations
- PubMed Links to PubMed
Recent Activity
- Addressing Risk for Waterborne Disease - Global Issues in Water, Sanitation, and... Addressing Risk for Waterborne Disease - Global Issues in Water, Sanitation, and Health
Your browsing activity is empty.
Activity recording is turned off.
Turn recording back on
Connect with NLM
National Library of Medicine 8600 Rockville Pike Bethesda, MD 20894
Web Policies FOIA HHS Vulnerability Disclosure
Help Accessibility Careers
- Open access
- Published: 24 July 2023
Severity of waterborne diseases in developing countries and the effectiveness of ceramic filters for improving water quality
- Godfrey Michael Shayo ORCID: orcid.org/0000-0001-6082-5897 1 ,
- Elianaso Elimbinzi 1 ,
- Godlisten N. Shao 1 &
- Christina Fabian 1
Bulletin of the National Research Centre volume 47 , Article number: 113 ( 2023 ) Cite this article
11k Accesses
28 Citations
Metrics details
It is anticipated that three (3) billion people will experience water stress by 2025 due to limited access to clean water. Water-related diseases and fatalities affect both industrialized and developing countries. Waterborne diseases are challenging worldwide, especially in developing countries. This article evaluates strategies used by various countries, particularly developing countries, to combat waterborne diseases. These strategies have been largely successful in reducing the prevalence of water-related diseases in developing countries.
Main body of the abstract
The effectiveness of these strategies is evaluated in terms of their ability to remove water contaminants such as bacteria, viruses, and chemicals. Different strategies can be used, including traditional water treatment techniques such as boiling, chlorination, flocculation, solar disinfection and ceramic-based water filtration systems. These methods can help improve water quality and safety. The choice of strategy depends on the specific contaminants in the water and the desired outcome. Proper implementation of these strategies is key to ensuring safe drinking water.
Short conclusion
It was revealed that in developing countries, multiple water treatment techniques are used. This has led to the reduction in waterborne diseases from 50 to 90%. Ceramic-based water purification systems are reportedly the modern and least expensive technique, since they are highly efficient and can be made locally. Thus, ceramic water filtration systems are widely used due to their affordability and easy maintenance.
Waterborne diseases are conditions caused by pathogenic microorganisms such as bacteria, protozoa, and viruses transmitted through water. When measures are delayed, these pathogens may cause adverse effects on human health, such as disability, illness, disorders, or death (Landrigan et al. 2020 ). Transmission of these pathogens occurs while using infected water for drinking, food preparation, and washing clothes (WHO 2022 ). However, most waterborne infections are spread by the fecal–oral pathway, which happens when human feces are consumed by drinking contaminated water or eating infected food, which is mostly caused by inadequate sewage management and sanitation. Waterborne pathogens, which accelerate waterborne diseases, significantly affect people’s health by causing mortality and morbidity (Ferreira et al. 2021 ; Gall et al. 2015a , b ; Shailemo et al. 2016 ). Waterborne diseases can cost people their lives and their socioeconomic status. Several research reports, government and non-government resources demonstrate this quietly. Access to clean water and sanitation facilities is essential for the prevention of waterborne diseases and the protection of public health. Proper management of water resources is critical for the prevention and control of waterborne diseases. Water quality monitoring and surveillance is necessary to protect public health.
Globally, 2.1 billion people lack access to clean and safe drinking water, resulting in 2.2 million deaths from waterborne diseases each year (UN 2019 ). Domestic water supplies must be free of disease-carrying microbes and other chemical contaminants to be safe for human consumption. It was once anticipated that until 2021, only 44% of the world’s population would have access to safe sources of water. This left a larger population, i.e., 56% of the world’s population, with access to unsafe and contaminated water from sewage, septic tanks, latrines, agricultural activities, and other human activities (World Health Organization 2020 ). Contamination of surface and groundwater ensures that waterborne diseases persist, particularly in developing countries. Currently, the global picture of water and health has a strong local dimension, with 1.1 billion people still lacking access to improved drinking water sources and 2.4 billion to adequate sanitation. There is extensive evidence that water-related, sanitation, and hygiene-related diseases account for 2.2 million deaths annually and an annual loss of 8.2 million disability-adjusted life (Anyango 2019 ; Kätzl 2019 ). The severity is much higher in developing countries than developed countries.
Waterborne diseases are one of society's most persistent and economically disastrous biological threats. Four-fifths of all illnesses in developing countries are caused by waterborne diseases, with diarrhea being the leading cause of childhood deaths (Luby et al. 2018 ). Generally, 1.8 million people die every year from waterborne diseases including cholera, typhoid, urinary tract infections, schistosomiasis and other diarrheal diseases. Nevertheless, waterborne diarrhea remains a prominent cause of mortality and sickness among children in developing nations, with 90% of diarrhea fatalities occurring in children under five. Rural residents in developing countries use discharge near or around neighboring shrubs and jungles for defecation, which results in fecal pollution of water in rural African and other developing-country locations. (Manetu and Karanja 2021 ). Common waterborne diseases include bacteria-caused diseases such as cholera, typhoid, and diarrhea, protozoa such as amoebiasis, and viral diseases such as retrovirals, hepatitis A, hepatitis E, and polio infections.
In contrast to many other outbreaks of diseases with incurable diagnoses or expensive preventions and treatments (Paliwal 2021 ), waterborne infections can be combated with local, affordable resources, minimal lifestyle changes, culturally relevant solutions, and clear and affordable awareness campaigns. Due to their ambiguity and variable applicability to different societies, environments, and durations, these sorts of solutions are called acceptable strategies. This paper reviews several strategies on their efficacy in combating waterborne diseases, particularly in rural regions of developing countries. Researchers have reported on several different strategies previously. A number of suggestions are provided, especially for developing countries that still suffer the brunt of waterborne disease. Finally, it suggests cost-effective and easy strategies when employed.
Severity of waterborne diseases in the world, developing countries and rural areas
Waterborne infections are transmitted through infected drinking water and food sources. The major causes of contamination are poor hygiene and sanitation. According to the World Bank, 2.6 billion people worldwide lack access to basic sanitation, which is defined as a clean and safe toilet or latrine (Gall et al. 2015a , b ; Weststrate et al. 2019 ). As a result, more than a quarter of the world's population must defecate behind buildings, in fields, or near communal water supplies. Disease transmission is significant when fecal matter is not properly disposed of. Infection and sickness can result from unintentional contact with excrement by people or other living things like pets or flies. In addition, using untreated human waste as fertilizer in agricultural techniques results in many infectious diseases. Additionally, due to a lack of control over the movement and habitat of most animals, pollution of nearby water sources by the feces of both domesticated and wild animals is a significant issue that is frequently more challenging to manage (Diedrich et al. 2023 ) .
Around 15% of the world's population lives in water-stressed areas (Javed and Kabeer 2018 ). Rural areas in developing nations lack access to reliable clean water supply points. Thus, they are vulnerable to waterborne diseases (Gwenzi and Sanganyado 2019 ). On the other hand, around 2.5 billion people lack access to proper sanitation, and 2–2.5 million people die from diarrhea each year (Javed and Kabeer 2018 ). Therefore, most people in these places drink untreated water from readily available contaminated sources, putting them at risk of contracting waterborne diseases. Generally, contaminated water is commonly used as a medium for disease transmission (Shailemo et al. 2016 Ali and Ahmad 2020 ).
The prevalence of waterborne intestinal pathogens such as bacteria, viruses and protozoa in domestic water sources poses a serious health risk to humans (Wen et al. 2020 ). The majority of outbreaks, though infrequent, are usually associated with sewage-contaminated or inadequately treated water. Figure 1 illustrates the transmission of waterborne diseases in the human population. Contaminated water sources serve as the primary reservoir for various contaminants, including bacteria, viruses and chemicals. These contaminants can enter the human body through ingestion, inhalation, or contact with contaminated water. Inadequate sanitation and poor hygiene practices further facilitate the spread of waterborne diseases. Once inside the body, these pathogens can cause a range of illnesses, such as gastroenteritis, cholera, hepatitis, and parasitic infections. Effective prevention and control measures, such as access to clean drinking water, proper sanitation systems, education on hygiene practices and the employment of water treatment techniques such as filtration, are crucial for reducing the incidence and impact of waterborne diseases. By addressing these factors, we can safeguard public health and promote a safer and healthier environment. Furthermore, sewage system failure and overpopulation raise the danger of infectious disease transmission, either via the virtual presence of a large number of bacteria in the environment or through contaminated drinking water (Mwambete and Tairo 2018 ).
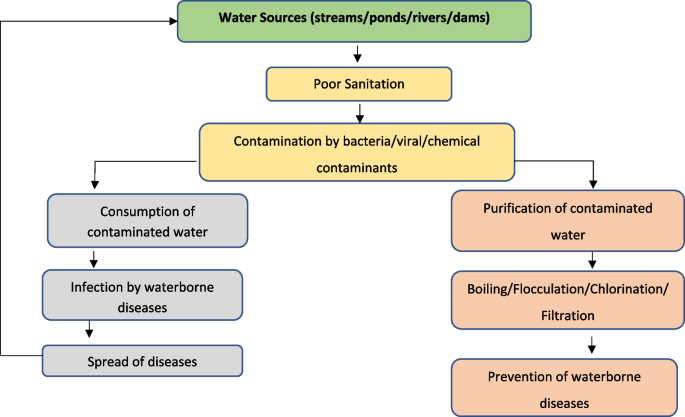
Schematic presentation of waterborne diseases transmission in human being
Although access to clean water is somehow managed in urban areas of developing countries, the situation is still poor or non-existent in rural parts of these countries (Murei et al. 2022 ). Approximately, 49% of unimproved sources, such as dug wells, natural springs, and other surface water sources are observed in rural areas. Diarrhea occurs worldwide and causes 4% of all deaths and 5% of disability loss. For example, in Bangladesh, 35 million people are daily exposed to elevated arsenic levels in their drinking water. This will ultimately threaten their health and shorten their life expectancy (World Health Organization 2020 ). Infection is common in low-income and middle-income countries with poor sanitary conditions and hygiene practices, where most children almost 90%, have been infected with the hepatitis A virus before 10 years, most often without symptoms (WHO 2022 ). Infection rates are low in high-income countries with proper sanitary and hygiene conditions.
Strategies in combating waterborne diseases
There are ways for disadvantaged people all over the world, especially those living in rural regions, to get access to clean water for drinking and other household needs. These may be referred to point-of-use (POU). Several domestic treatment methods, including boiling, sun disinfection, filtration, chemical disinfection like chlorination and flocculation, and/or sedimentation, have been implemented by several developing nations as part of their adaptation to treatment tactics (Branz et al. 2017 ; Lantagne and Yates 2018 ). The main treatment methods are shown in Table 1 along with each method's characteristics. People use these methods to prevent waterborne illnesses. Results for addressing various water pollutants, such as color, total solids, turbidity, and odor, are highly encouraging. However, in some cases, they cannot remove other water contaminants such as virus, chemicals that is, chlorine, heavy metals and other organic contaminants and bacteria contaminants. This leaves it up to researchers to investigate the efficacy of creating a ceramic filtration system with multiple capabilities for water purification. This includes the incorporation of nanomaterials like silver, copper, and gold to remove bacterial and pathogenic microorganisms. However, the incorporation of hydroxyapatite helps to remove heavy metal chemical contaminants and improve pores structure for correction of color, pH, turbidity, total dissolved solids and biological oxygen demagnetization.
Water quality and resource protection are still funded by international and non-governmental groups. Several cases of aquatic infectious diseases have been documented (Annan et al. 2018 ). Incorporating nanoparticles of noble metals into filtration technology seems to be a viable option. Some studies have reported the removal of viral and chemical contaminants through doping conventional ceramic water filters with metal oxide. Conventional ceramic water filters have been advantageous in the filtration of some water contaminants, such as bacteria, protozoa and other contaminants with ≥ 2 µm diameter size (Nigay et al. 2019 ). Recently, some studies have reported the removal of viruses through doping of standard ceramic water filters with metal oxides, such as aluminum oxide, magnesium oxide, iron oxide and titanium oxide (Mutuma et al. 2015 ; Nigay et al. 2019 ; Shao et al. 2014 , 2015 ) and chemical contaminants through hydroxyapatite (HA) doping (Haider et al. 2019 ; Nigay et al. 2019 ; Farrow et al. 2018 ). For a decade, the filtration of water contaminants such as physical, chemical and biological contaminants has been in practice in several countries. This is to address the problem of lack of safe and clean drinking water.
Viral waterborne diseases in developing countries
Viruses are the tiniest microorganisms of all parasites, with an approximate size ranging from 0.03 to 0.1 µm. Viruses are present in drinking water sources but their impact on human health is less widely understood and acknowledged. However, swallowing them can have major health consequences (Gall et al. 2015a , b ; Adelodun et al. 2021 ). More than 100 different human and animal enteric viruses have been identified as water transmissible. Rotavirus, enterovirus, norovirus and hepatitis A and E are all viral infections spread through water. Researchers have had limited success in deactivating or eliminating viruses from drinking water (Annan et al. 2018 ). Surface water contamination with enteric viruses due to human waste disposal is a public health hazard. This is especially true if these surface waterways are used for recreational, irrigation or drinking water production (Gall et al. 2015a , b ; McKee and Cruz 2021 ). Polluted water transfers viruses, including drinking and recreational water. Outbreaks involving huge numbers of diseased people are typical because numerous people may ingest a batch of water or come into contact with contaminated materials (McKee and Cruz 2021 ). Viral gastroenteritis outbreaks are mostly caused by norovirus, whereas viral hepatitis outbreaks are mostly caused by Hepatitis A Virus and rarely by Hepatitis E Virus (Bosch et al. 2011 ; McKee and Cruz 2021 ).
Viral infections, particularly those caused by rotavirus, are the most common causes of acute diarrheal diseases. Over half a million people worldwide die each year from the rotavirus, which is so pervasive that it infects almost every child by the age of five (Charoenwat et al. 2022 ). Typically, viral hepatitis affects the liver. It can be acute (fresh infection, fast onset) or chronic (long onset) (Aggarwal 2011 ; Kim et al. 2021 ). Infection with one of the five known hepatotropic viruses (hepatitis A, B, C, D and E viruses) causes viral hepatitis. Viral-based waterborne diseases can also be transmitted through inhalation or contact with skin and eyes which can both spread viruses, resulting in respiratory and ocular diseases. For healthy people, viral infections are typically self-limiting, but in children under five, the elderly, immune-compromised adults and pregnant women, they are at higher risk (Gall et al. 2015a , b ). Waterborne virus-based infections may be more common in developing countries, where hunger is common, and there are huge populations of HIV-positive (Gall et al. 2015a , b ; WHO 2022 ).
For this paper, only waterborne hepatitis viruses A and E will be discussed. In populations with unsafe water and inadequate sanitation, viral hepatitis A and E are food and waterborne diseases that can cause acute epidemics. They do not cause chronic infection or liver damage, and there is no treatment for them. Improvements in sanitation, food safety, and immunization are all effective prevention methods (Aggarwal 2011 ; Kim et al. 2021 ). The most typical clinical outcome of hepatitis A or E virus infection is a sickness typified by an abrupt onset of fever and systemic symptoms, followed by jaundice a few days later.
Hepatitis A and E viral waterborne diseases
Hepatitis A is a self-limiting liver illness caused by Hepatitis A virus infection. Hepatitis A viral infection spreads by the fecal–oral route, which can be transmitted directly from person to person or indirectly through the intake of feces-contaminated food or water (Foster et al. 2019 ). Because the hepatitis A virus is abundantly discharged in feces and may live in the environment for extended periods of time, it is usually a food-waterborne illness (Foster et al. 2019 ; Gullón et al. 2017 ). In regions where sanitation is inadequate and living conditions are dense, infections arise early in life. Infections are delayed due to increased sanitation and hygiene, and the number of people vulnerable to the disease rises (Gullón et al. 2017 ). In these circumstances, fecal contamination from a single source might result in explosive epidemics. Adults are increasingly contracting hepatitis A virus infections in most developed countries, where hepatitis A is no longer considered a childhood illness (Foster et al. 2019 ; Gullón et al. 2017 ).
Hepatitis E is an acute hepatitis caused by the Hepatitis E Virus infection. The virus spreads predominantly by the fecal–oral route, and it is extremely prevalent in certain underdeveloped nations where drinking water might be contaminated (Aggarwal 2011 ; Magana-Arachchi and Wanigatunge 2020 ). It manifests itself as outbreaks and occasional instances of acute hepatitis in these highly endemic locations. The illness is usually self-limiting and resembles other hepatotropic viruses. However, in some cases, the condition progresses to severe liver failure (Magana-Arachchi and Wanigatunge 2020 ). The Indian subcontinent, China, Southeast and Central Asia, the Middle East and northern and western Africa are all highly endemic to hepatitis E (Yekta et al. 2021 ). Hepatitis E outbreaks of various magnitudes have been documented in these regions. Furthermore, hepatitis E virus infection is responsible for a substantial number of sporadic acute hepatitis cases in these locations. The most prevalent mode of illness transmission in these places is water (Yekta et al. 2021 ). The hepatitis E virus has been linked to a 25% mortality rate in pregnant women (World Health Organization 2022 ). Several strategies have been discussed to combat hepatitis A and E viral waterborne infections including physical elimination, chemical treatment and UV light disinfection.
Strategies for combating viral waterborne diseases in developing countries
In the elimination of viral water contaminants from drinking water, several strategies have been used. However, there are two common and effective strategies used in the world and particularly in developed countries, which are physical elimination of pathogens by conventional treatment and the inactivation of viral pathogens using ultraviolet irradiation or chemical oxidants such as chlorine, chloramines, ozone and chlorine dioxide (Gall et al. 2015a , b ). Because viruses are so small, conventional treatment methods, such as filtration, are unsuccessful in physically eliminating them (Gall et al. 2015a , b ; Nigay et al. 2019 ). Disinfectants are heavily dependent on water chemistry and local restrictions. A common disinfection technique in recent years has been chlorination, where free chlorine is derived from hypochlorous acid and hypochlorite ions that are dissolved in water and hydrolyzed. This strategy has been used to disinfect water since the early 1900s (Branz et al. 2017 ; Gall et al. 2015a , b ; Lantagne and Yates 2018 ). This powerful oxidant renders most viruses dormant. However, free chlorine treatment may release harmful disinfection by-products and fails to control Cryptosporidium, a protozoan that causes diarrhea and spreads through water (Khan et al. 2019 ; Gall et al. 2015a , b ). To control the formation of regulated toxic disinfection by-products, some drinking water utilities are switching to monochloramine which is formed by mixing chlorine and ammonia with the latter in slightly excess; and/or either monochromatic (254 nm) or polychromatic (200–300 nm) ultraviolet (UV) light to control both disinfection by-products formation and Cryptosporidium contamination. In spite of these modifications to the disinfection method, the UV light technique comes with a very high cost for virus control compared to other conventional methods (Gall et al. 2015a , b ; Ibrahim et al. 2021 ).
In order to deal with the viral-based waterborne situation, total abstinence from all water sources such as streams, ponds, rivers and lakes is necessary, as well as other water sources that may be contaminated by waterborne pathogens and other chemicals. With a variety of methods, some developed countries, such as the United States, Canada, the Netherlands, and Western Australia, have shown efficiency in wastewater treatment. This is due to differences in socioeconomic factors (Ferreira et al. 2021 ). Most waterborne illnesses are not prevalent in developed countries because of sophisticated water systems that filter and chlorinate water to eradicate all disease-carrying organisms. In developing countries, however, waterborne diseases such as Hepatitis A and E, remain prevalent. The strategies employed in developed countries may not be feasible, particularly in rural areas where proper sanitation and infrastructure for water management are difficult to attain (Levy et al. 2018 ). As a result, this review recommends using point-of-use water treatment technology as a replacement, particularly for ceramic water filters that can be produced at a price affordable for rural residents when doped with metal oxides like alumina, titania, iron oxide, zinc oxide, or magnesium oxide (Mutuma et al. 2015 ; Nigay et al. 2019 ).
Bacterial waterborne diseases
Bacteria are single-celled or non-cellular, spherical, spiral or rod-shaped microorganisms that reproduce by fission and are key pathogens and biochemical characteristics. Bacteria are well-known diarrhea-causing diseases transmitted through contaminated drinking water. Depending on the bacteria kind and number present, these bacteria may or may not be detrimental, but the cumulative effect might be devastating. Bacteria are generally between 0.5 and 2 µm long (Annan et al. 2018 ). Vibrio cholerae , Salmonella sp., Campylobacter sp., Shigella sp., and Staphylococcus aureus are all bacteria spread through water. Coliform bacteria are a group of microorganisms found in the environment and mammals' intestines. They are usually harmless, but their presence indicates that drinking water's microbiological quality is of concern (Mwambete and Tairo 2018 ; World Health Organization 2006 ). Some coliforms bacteria include Escherichia, Serratia, Enterobacter, Proteus, Klebsiella, Citrobacter, Yersinia and Hafnia species . However, E. coli is the only member found in the intestines of mammals including humans; thus, its presence indicates recent fecal contamination and the possible presence of other waterborne pathogens.
Drinking water is a significant vehicle for bacterial waterborne infections such as cholera, diarrhea and typhoid fever (Gwenzi and Sanganyado 2019 ; Mwambete and Tairo 2018 ; World Health Organization 2006 ). Cholera is caused by the bacteria Vibrio cholerae , which causes severe diarrhea, vomiting, dehydration and death. It can be severe if not treated properly, up to 50% of the time. However, medication can reduce the severity to as little as 1% of the time. Cholera causes 100,000 deaths worldwide (Lee et al. 2017 ). Salmonella typhi bacteria are the source of the potentially fatal bacterial infection known as typhoid fever. There are still roughly 21 million cases of typhoid fever each year in developing nations. Only people carry Salmonella typhi . Typhoid fever patients have bacteria in their blood and intestines. Few people, called carriers, recover from typhoid fever but still carry the germ. Sick persons and carriers excrete S. typhi in their stools. Consuming or drinking food or beverages that have been touched by someone shedding S. typhi bacteria or drinking or washing food with sewage contaminated with S. typhi bacteria can result in typhoid fever (Brockett et al. 2020 ).
In developing countries, E. coli is the most common cause of diarrheal disease infections and human gastrointestinal tract infections caused by ingesting contaminated water (Gwenzi and Sanganyado 2019 ). In Africa, for instance, a severe cholera epidemic broke out in Zimbabwe in 2008 and quickly spread to neighboring nations (Zambia, Botswana, Mozambique and South Africa). Due to poor sanitation and waste management practices and a limited supply of clean piped water, the scarcity of safe drinking water in Zimbabwe's urban areas had a significant role in the development and spread of the disease. Poor water sanitation and hygiene are linked to a higher proportion of intestinal parasitic infections, with the majority being fecal–oral (Gwenzi and Sanganyado 2019 ; Gwimbi et al. 2019 ). In rural regions of most developing nations, where water supplies are communally shared and exposed to many fecal–oral transmission paths within their neighborhood boundaries, bacterial contamination of drinking water is a major contributor to waterborne illnesses (Reece et al. 2017 ; Iwu and Okoh 2019 ). E. coli infections linked to polluted water continue to be a serious public health problem, as their presence indicates the prevalence of deadly disorders such as diarrhea (Iwu and Okoh 2019 ). Despite the fact that the endemicity and intensity of bacterial waterborne illnesses have decreased in developing countries, the case fatality rates in cholera cases remain significantly higher in Africa (about 60%) than in Asia (29%) (Montufar-Salcedo, 2018 ). However, the World Health Organization (WHO) reports that 1.3 million suspected cases of typhoid fever have been recorded in Africa since 2021, with 502 deaths (2%) out of 30,934 confirmed cases in DRC. These are the most common bacterial-associated waterborne diseases in most developing countries (Gwimbi et al. 2019 ).
Strategies for combating bacterial waterborne diseases
Recently, bacterial-based water contaminants have been solved thanks to the availability of common point-of-use water treatment technologies. In most developing countries, the technologies include boiling, chlorination of contaminated water, solar disinfection and filtration techniques such as bone char, bio-sand, slow sand, membrane purifiers and ceramic filters (Farrow et al. 2018 ). Although all of them work effectively in bacterial removal, ceramic filters are perceived by most users and developers due to their easy and affordable cost of fabrication, as they require the availability of regional materials such as clay, soil, sawdust, starch, wheat flour, and milled rice husk which hence makes their dissemination to people cost-effective and economically sound. The incorporation of noble metals into ceramic water filters ensures the efficient functioning or performance of the filters, this is to say, by increasing bacterial disinfection or by increasing bio-film disinfection ability. Metal oxide nanoparticles' antibacterial capabilities, manufacturing techniques and microorganisms removed during water treatment are summarized in Table 2 .
From Table 2 , based on the different Lewis-dot structures, metal oxides display diverse physicochemical and functional properties, including magnetic, optical, mechanical, and electrical properties/features (Raghunath and Perumal 2017 ). They have shown the ability to interact with bacteria through electrostatic interactions through prokaryotic cell walls and enzyme or DNA alteration through reactive oxygen species (ROS) production (Gold et al. 2018 ). Under light exposure (He et al. 2016 ), magnesium oxide nanoparticles act as antibacterial agents and produce ROS. The ROS then enters the bacterial cell membrane while reducing both oxidative stresses on the cell organelles and lipid peroxidation, thereby preventing oxidative degradation of lipids (Gold et al. 2018 ). Since titania is a strong photocatalytic material with high oxidizing power and long-term stability, it can generate ROS with a wavelength of around 320–385 nm, hence its ability as an antimicrobial agent (Kumaravel et al. 2021 ). The action of metal oxide antimicrobial agents involves several working mechanisms, including cell membrane damage due to electrostatic interaction, disruption in metal/metal ion homeostasis, production of ROS and oxidative stress, protein and enzyme dysfunction, genotoxicity, signal transduction inhibition, and photo-removal (Raghunath and Perumal 2017 ).
However, from Table 2, it is anticipated that a higher concentration of MgO inhibits bacteria’s growth against E. coli which is higher than Bacillus sp . On the other hand, CuO provides more room to be used as a biocidal agent, such as against B. subtilis. This is due to its cost-effectiveness and better biocidal ability than other noble metal oxides (Hoseinnejad et al. 2018 ). In many studies, ZnO is proposed to have higher antibacterial ability than other metal oxides since they can pose a threat to both gram-positive and gram-negative bacteria. Furthermore, Al 2 O 3 at high concentrations has mild deactivation properties owing to the free radical scavenging capability of nanoparticles that prevent cell wall disintegration (Makvandi et al. 2020 ). Nevertheless, Al 2 O 3 has also been suggested to trap viral contaminants due to its positively charged surface (Nigay et al. 2019 ).
Chemical contamination of water
Water is a carrier of infectious microorganisms such as bacteria, parasites and viruses that spread via the fecal–oral route in water-based diseases. Similarly, chemicals are sometimes thought to be a source of infectious agents (Javed and Kabeer 2018 ). Water-stressed areas are home to about 15% of the world's population. Waterborne diseases are caused by chemical toxins, mostly found in industrial, municipal, and agricultural wastes (Javed and Kabeer 2018 ). For instance, heavy metals such as chromium, cadmium, nickel, lead, mercury and arsenic; cations, such as sodium, potassium, and calcium; anions, such as carbonates, bicarbonates, and nitrates; and pesticides, such as dichlorodiphenyltrichloroethane and benzene hexachloride enter water bodies from point and non-point sources and cause several health complications among people in many developing countries (Syafrudin et al. 2021 ).
Pesticide use has a number of advantages, including better food quality and quantity and reduced insect-borne diseases, but it has also prompted concerns about potential negative impacts on the environment, especially water sources (Syafrudin et al. 2021 ). Pesticides end up in bodies of water due to runoff from agricultural fields and industrial waste. Soluble pesticides are taken away by water molecules, which percolate lower into the soil layers and eventually reach surface waters and groundwater (Syafrudin et al. 2021 ). As a result, water quality deteriorates and drinkable water quantity decreases. Drinking water contaminated with heavy metals, pesticides, cations, and anions causes life-threatening complications in the gastrointestinal, renal, cardiovascular, pulmonary, and reproductive systems (Syafrudin et al. 2021 ). Furthermore, chemicals carried by polluted water can cause urinary tract burning and calculi, leukomelanosis, hyperkeratosis, black foot disease, neuropathy and cancer (Javed and Kabeer 2018 ; Syafrudin et al. 2021 ).
Chemicals in drinking water that exceed allowable levels may harm human health. This could be caused by human activities or natural occurrences. Chemical pollutants in drinking water have also been linked to a wide range of negative health impacts, including cancer, cardiovascular illness, neurological disease, and miscarriages. Leaching, spills, runoff, and air deposition are ways through which chemicals enter water systems (Annan et al. 2018 ). Heavy metals are found naturally in the earth's crust and are long-lasting environmental pollutants since they cannot be degraded or removed. They enter the human system in tiny amounts from food, air, and water, and bioaccumulate over time (Ali et al. 2017 ; Annan et al. 2018 ). Table 3 shows WHO and USEPA maximum permissible heavy metals in drinking water values.
With an acceptable concentration of 0.002 mg/L poisonous level, thallium and mercury are the most poisonous metals (Table 3 ). This puts human health at high risk compared to all other metals mentioned in the table. While nitrate, with a WHO rating of 11.3 mg/L and a USEPA rating of 10.0 mg/L, represents the highest allowable chemical concentration in the human body. Chemical contaminants in drinking water can pose a threat to human health sometimes, but the human body needs several heavy metal elements in their divalent cation forms, such as Zn 2+ , Fe 2+ and Cu 2+ . For instance, these metal divalents are required by the human body in the regulation of numerous physiological functions. These functions include protein and nucleic acid synthesis, antioxidant defense, and membrane stabilization. However, these metal divalents are required by the human body at very low concentration (Ali et al. 2017 ; Rehman et al. 2021 ). If their concentration exceeds the body's requirement level, metal divalent leads to health effects (Ali et al. 2017 ). Other heavy metals are poisonous to humans, such as Cd 2+ , Pb 2+ , Co 2+ , Pt 2+ and Ni 2+ . When the human body is contaminated with these metals, the kidneys, for instance, suffer the most. Hence, several effects are observed, including a decrease in essential elements entry due to heavy metal competition (Ali et al. 2017 ; Rehman et al. 2021 ).
Strategies for combating chemical contamination of water
Several studies have reported some positive progress advances in the discovery of therapeutic tools, such as cell protectors and metal chelators. These tools can be administered when an individual has taken the chemicals in any way, particularly through contaminated drinking water. But treatment must be a last option if, at all costs, the situation can be prevented from happening. Studies have reported developing point-of-use water treatment technologies, such as ceramic water filters, among many others as speculated in Table 1 , being more feasible for many people due to their low cost and ease of fabrication (Gupta et al. 2018 ; Farrow et al. 2018 ). Ceramic filters can be boosted in their efficiency in the removal of heavy metals, pesticides, and organic chemical contaminants when doped with hydroxyapatite chemicals, and the chemical materials made from bones (Haider et al. 2019 ; Nigay et al. 2019 ; Farrow et al. 2018 ). Nigay et al. ( 2019 ) reported that through a substitution mechanism, HA chemicals can interchange their chemical contents, that is, calcium ions, hydroxyl groups, and phosphate groups, with the heavy metal chemicals present in the contaminated water (Nigay et al. 2019 ).

Future prospect
Ceramic water filters, as used in many developed countries such as the USA, Netherlands, Canada, and Western Australia, can be used in developing countries with some modifications. This will improve performance and efficiency at the point-of-use. Conventional ceramic water filters can improve water quality in several parameters but fail in others. For instance, most bacterial contaminants can be physically filtered through conventional ceramic water filters. However, after some time of filter use, bacteria and mold grow on the surface of the system. Incorporating ceramic water filters with noble metals such as silver, copper, or gold in their nanoparticle form removes bacteria and prevents the system from becoming infected with protozoa (Loza et al. 2020 ; Praveena and Aris 2015 ). However, for several years, viral-based contaminants have been linked to hepatitis A and E diseases, which may cause liver cancer if chronic. Removal of viruses is quite challenging due to their small size, so they cannot be removed through physical strains. However, doping ceramic water filters with metal oxides including titania, alumina, magnesium oxide, or iron oxide facilitates the adsorption of viruses from water (Haider et al. 2019 ; Mutuma et al. 2015 ; Shao et al. 2015 ). This is due to the fact that viruses have negative surface charges and hence can be attracted to metal oxides, which are positively charged. Additionally, chemicals can be removed from water by hydroxyapatite chemicals (Haider et al. 2019 ; Nigay et al. 2019 ; Farrow et al. 2018 ). Doping ceramic water filters with hydroxyapatite is feasible and increases chemical removal efficiency. Therefore, the feasibility of having one system that simultaneously removes bacterial, viral, and chemical contaminants is quite possible. This is when a ceramic water filter is incorporated with noble metal nanoparticles and doped with metal oxides and hydroxyapatite.
Conclusions
Regardless of the disinfection method employed by a drinking water utility, cross-contamination can happen throughout the water distribution infrastructure. This is due to cavitation and unintended depressurization when treated water moves from the treatment facility to the point-of-use. However, because municipal water services are typically not available in poor nations, residents must acquire water from other nearby sources. Most of these sources are tainted with pollutants and bacteria that cause waterborne illness. The World Health Organization estimated in 2017 that environmental changes including expanding access to clean drinking water and raising sanitation and hygiene standards may prevent 94% of cases of waterborne diarrhea diseases. However, the increasing water availability, sanitation, hand washing, and domestic water treatment and safe storage can reduce diarrhea episodes by 25%, 32%, 45% and 39%, respectively. Although, these distribution systems need additional disinfectants. This review also offers recommendations for how developing nations can lower waterborne illnesses prevalence. These include raising the quantity and quality of drinking water, ensuring safe sewage disposal, and offering accessible, affordable sanitation solutions. For example, the adoption of point-of-use water treatment technologies. These technologies are simple, low-cost, and have the potential to reduce waterborne illnesses significantly. Furthermore, these solutions should be combined with educational campaigns to ensure that people are aware of how to use and maintain the technologies.
Availability of data and materials
Not applicable.
Abbreviations
Reactive oxygen species
Escherichia coli
United State Environmental Protection Agency
World Health Organization
Point of use
Ceramic water filters
Human immunodeficiency virus
Hydroxyapatite
Ultraviolet light
Hepatitis A viruses
Hepatitis E viruses
United Nation
Adelodun B, Ajibade FO, Ighalo JO, Odey G, Ibrahim RG, Kareem KY et al (2021) Assessment of socioeconomic inequality based on virus-contaminated water usage in developing countries: a review. Environ Res 192:110309
Article CAS PubMed Google Scholar
Aggarwal R (2011) Clinical presentation of hepatitis E. Virus Res 161(1):15–22. https://doi.org/10.1016/j.virusres.2011.03.017
Aggarwal R, Naik S (2009) Epidemiology of hepatitis E: current status. J Gastroenterol Hepatol 24(9):1484–1493. https://doi.org/10.1111/j.1440-1746.2009.05933.x
Article PubMed Google Scholar
Ali A, Ahmed A, Gad A (2017) Chemical and microstructural analyses for heavy metals removal from water media by ceramic membrane filtration. Water Sci Technol 75(2):439–450. https://doi.org/10.2166/wst.2016.537
Ali SA, Ahmad A (2020) Analysing water-borne diseases susceptibility in Kolkata Municipal Corporation using WQI and GIS based Kriging interpolation. GeoJournal 85(4):1151–1174
Article Google Scholar
Annan E, Agyei-Tuffour B, Bensah YD, Konadu DS, Yaya A, Onwona-Agyeman B, Nyankson E (2018) Application of clay ceramics and nanotechnology in water treatment: a review. Cogent Eng 5(1):1476017
Anyango MJ (2019) Water, sanitation and hygiene practices as predictors of diarrhoea occurrence among school age children in Ganze Sub County, Kenya. 206
Azam A, Ahmed AS, Oves M, Khan MS, Habib SS, Memic A (2012) Antimicrobial activity of metal oxide nanoparticles against Gram-positive and Gram-negative bacteria: a comparative study. Int J Nanomed. https://doi.org/10.2147/IJN.S35347
Bitew BD, Gete YK, Biks GA, Adafrie TT (2018) The effect of SODIS water treatment intervention at the household level in reducing diarrheal incidence among children under 5 years of age: a cluster randomized controlled trial in Dabat district, northwest Ethiopia. Trials 19(1):412. https://doi.org/10.1186/s13063-018-2797-y
Article PubMed PubMed Central Google Scholar
Bosch A, Sánchez G, Abbaszadegan M, Carducci A, Guix S, Le Guyader FS, Netshikweta R, Pintó RM, van der Poel WHM, Rutjes S, Sano D, Taylor MB, van Zyl WB, Rodríguez-Lázaro D, Kovač K, Sellwood J (2011) Analytical methods for virus detection in water and food. Food Anal Methods 4(1):4–12. https://doi.org/10.1007/s12161-010-9161-5
Branz A, Levine M, Lehmann L, Bastable A, Ali SI, Kadir K, Yates T, Bloom D, Lantagne D (2017) Chlorination of drinking water in emergencies: a review of knowledge to develop recommendations for implementation and research needed. Waterlines 36(1):4–39. https://doi.org/10.3362/1756-3488.2017.002
Brockett S, Wolfe MK, Hamot A, Appiah GD, Mintz ED, Lantagne D (2020) Associations among water, sanitation, and hygiene, and food exposures and typhoid fever in Case-Control studies: a systematic review and meta-analysis. Am J Trop Med Hyg 103(3):1020
Bui XT, Nguyen PT, Nguyen VT, Dao TS, Nguyen PD (2020) Microplastics pollution in wastewater: characteristics, occurrence and removal technologies. Environ Technol Innov 19:101013
Bulta AL, Micheal GAW (2019) Evaluation of the efficiency of ceramic filters for water treatment in Kambata Tabaro zone, southern Ethiopia. Environ Syst Res 8(1):1. https://doi.org/10.1186/s40068-018-0129-6
Burleson G, Tilt B, Sharp K, MacCarty N (2019) Reinventing boiling: A rapid ethnographic and engineering evaluation of a high-efficiency thermal water treatment technology in Uganda. Energy Res Soc Sci 52:68–77
Charoenwat B, Suwannaying K, Paibool W, Laoaroon N, Sutra S, Thepsuthammarat K (2022) Burden and pattern of acute diarrhea in Thai children under 5 years of age: a 5-year descriptive analysis based on Thailand National Health Coverage (NHC) data. BMC Public Health 22(1):1–10. https://doi.org/10.1016/j.erss.2019.02.009
Diedrich A, Sivaganesan M, Willis JR, Sharifi A, Shanks OC (2023) Genetic fecal source identification in urban streams impacted by municipal separate storm sewer system discharges. PLoS ONE 18(1):e0278548
Article CAS PubMed PubMed Central Google Scholar
El-Taweel GE, Ali GH (2000) Evaluation of roughing and slow sand filters for water treatment. Water Air Soil Pollut 120(8):21–28.
Article ADS CAS Google Scholar
Farrow C, McBean E, Huang G, Yang A, Wu Y, Liu Z, Li Y (2018) Ceramic water filters: a point-of-use water treatment technology to remove bacteria from drinking water in Longhai City, Fujian Province. China J Environ Inf 32(2):63–68
Google Scholar
Ferreira DC, Graziele I, Marques RC, Gonçalves J (2021) Investment in drinking water and sanitation infrastructure and its impact on waterborne diseases dissemination: The Brazilian case. Sci Total Environ 779:146279. https://doi.org/10.1016/j.scitotenv.2021.146279
Article ADS CAS PubMed Google Scholar
Fewtrell L, Kaufmann RB, Kay D, Enanoria W, Haller L, Colford JM (2005) Water, sanitation, and hygiene interventions to reduce diarrhoea in less developed countries: a systematic review and meta-analysis. Lancet Infect Dis 5(1):42–52. https://doi.org/10.1016/S1473-3099(04)01253-8
Foster MA, Hofmeister MG, Kupronis BA, Lin Y, Xia G-L, Yin S, Teshale E (2019) Increase in hepatitis A virus infections—United States, 2013–2018. MMWR Morb Mortal Weekly Rep 68(18):413–415. https://doi.org/10.15585/mmwr.mm6818a2
Gall AM, Mariñas BJ, Lu Y, Shisler JL (2015a) Waterborne viruses: a barrier to safe drinking water. PLoS Pathog 11(6):e1004867. https://doi.org/10.1371/journal.ppat.1004867
Gall AM, Shisler JL, Mariñas BJ (2015b) Analysis of the viral replication cycle of adenovirus serotype 2 after inactivation by free chlorine. Environ Sci Technol 49(7):4584–4590. https://doi.org/10.1021/acs.est.5b00301
Ghernaout D (2014) Coagulation and chlorination of NOM and algae in water treatment: a review. Int J Environ Monit Anal 2(6):23. https://doi.org/10.11648/j.ijema.s.2014020601.14
Ghernaout D (2017) Water treatment chlorination: an updated mechanistic insight review. Chem Res J 2:125–138
CAS Google Scholar
Gold K, Slay B, Knackstedt M, Gaharwar AK (2018) Antimicrobial activity of metal and metal-oxide based nanoparticles. Adv Ther 1(3):1700033. https://doi.org/10.1002/adtp.201700033
Article CAS Google Scholar
Gullón P, Varela C, Martínez EV, Gómez-Barroso D (2017) Association between meteorological factors and hepatitis A in Spain 2010–2014. Environ Int 102:230–235. https://doi.org/10.1016/j.envint.2017.03.008
Gupta S, Satankar RK, Kaurwar A, Aravind U, Sharif M, Plappally A (2018) Household production of ceramic water filters in western Rajasthan, India. Int J Serv Learn Eng Humanit Eng Soc Entrep 13(1):53–66. https://doi.org/10.24908/ijsle.v13i1.11150
Gwenzi W, Sanganyado E (2019) Recurrent Cholera Outbreaks in Sub-Saharan Africa: moving beyond epidemiology to understand the environmental reservoirs and drivers. Challenges 10(1):1. https://doi.org/10.3390/challe10010001
Gwimbi P, George M, Ramphalile M (2019) Bacterial contamination of drinking water sources in rural villages of Mohale Basin, Lesotho: exposures through neighbourhood sanitation and hygiene practices. Environ Health Prev Med 24(1):33. https://doi.org/10.1186/s12199-019-0790-z
Haider MS, Shao G, Ahmad A, Imran SM, Abbas N, Abbas G, Hussain M, Kim HT (2019) Facile, single-pot preparation of nanoporous SiO 2 particles (carrier) with AgNPs at core and crust for controlled disinfectant release. J Saudi Chem Soc 23(7):828–835. https://doi.org/10.1016/j.jscs.2019.02.005
He Y, Ingudam S, Reed S, Gehring A, Strobaugh TP, Irwin P (2016) Study on the mechanism of antibacterial action of magnesium oxide nanoparticles against foodborne pathogens. J Nanobiotechnology 14(1):54. https://doi.org/10.1186/s12951-016-0202-0
Hoseinnejad M, Jafari SM, Katouzian I (2018) Inorganic and metal nanoparticles and their antimicrobial activity in food packaging applications. Crit Rev Microbiol 44(2):161–181. https://doi.org/10.1080/1040841X.2017.1332001
Ibrahim Y, Ouda M, Kadadou D, Banat F, Naddeo V, Alsafar H et al (2021) Detection and removal of waterborne enteric viruses from wastewater: a comprehensive review. J Environ Chem Eng 9(4):105613
Iwu CD, Okoh AI (2019) Preharvest transmission routes of fresh produce associated bacterial pathogens with outbreak potentials: a review. Int J Environ Res Public Health 16(22):4407
Javed A, Kabeer A (2018) Enhancing waterborne diseases in pakistan & their possible control. Am Acad Sci Res J Eng Technol Sci 49(1):248–256
Jeon I, Ryberg EC, Alvarez PJ, Kim JH (2022) Technology assessment of solar disinfection for drinking water treatment. Nat Sustain 5(9):801–808
Kallman EN, Oyanedel-Craver VA, Smith JA (2011) Ceramic filters impregnated with silver nanoparticles for point-of-use water treatment in rural Guatemala. J Environ Eng 137(6):407–415. https://doi.org/10.1061/(ASCE)EE.1943-7870.0000330
Kätzl K (2019) Anaerobic biochar filtration of municipal raw sewage for wastewater reuse (Doctoral dissertation, Ruhr-Universität Bochum)
Kiagho B, Machunda R, Hilonga A, Njau K (2016) Performance of water filters towards the removal of selected pollutants in Arusha, Tanzania. Tanzan J Sci 42(1):134–147
Kim JU, Ingiliz P, Shimakawa Y, Lemoine M (2021) Improving care of migrants is key for viral hepatitis elimination in Europe. Bull World Health Organ 99(4):280–286. https://doi.org/10.2471/BLT.20.260919
Khan A, Shams S, Khan S, Khan MI, Khan S, Ali A (2019) Evaluation of prevalence and risk factors associated with Cryptosporidium infection in rural population of district Buner, Pakistan. PLoS ONE 14(1):e0209188
Kumaravel V, Nair KM, Mathew S, Bartlett J, Kennedy JE, Manning HG et al (2021) Antimicrobial TiO 2 nanocomposite coatings for surfaces, dental and orthopaedic implants. Chem Eng J 416:129071
Landrigan PJ, Stegeman JJ, Fleming LE, Allemand D, Anderson DM, Backer LC, Rampal P (2020) Human health and ocean pollution. Ann Glob Health 86(1):151
Lantagne D, Klarman M, Mayer A, Preston K, Napotnik J, Jellison K (2010) Effect of production variables on microbiological removal in locally-produced ceramic filters for household water treatment. Int J Environ Health Res 20(3):171–187. https://doi.org/10.1080/09603120903440665
Lantagne D, Yates T (2018) Household water treatment and cholera control. J Infect Dis 218(suppl_3):S147–S153. https://doi.org/10.1093/infdis/jiy488
Lee EC, Kelly MR, Ochocki BM, Akinwumi SM, Hamre KES, Tien JH, Eisenberg MC (2017) Model distinguishability and inference robustness in mechanisms of cholera transmission and loss of immunity. J Theor Biol 420:68–81. https://doi.org/10.1016/j.jtbi.2017.01.032
Article ADS MathSciNet PubMed PubMed Central MATH Google Scholar
Levy K, Smith SM, Carlton EJ (2018) Climate change impacts on waterborne diseases: moving toward designing interventions. Curr Environ Health Rep 5(2):272–282. https://doi.org/10.1007/s40572-018-0199-7
Li Y, Li J, Ding J, Song Z, Yang B, Zhang C, Guan B (2022) Degradation of nano-sized polystyrene plastics by ozonation or chlorination in drinking water disinfection processes. Chem Eng J 427:131690
Loomis D, Sobsey MD, Brown J (2008) Local drinking water filters reduce diarrheal disease in cambodia: a randomized, controlled trial of the ceramic water purifier. Am J Trop Med Hyg 79(3):394–400. https://doi.org/10.4269/ajtmh.2008.79.394
Loza K, Heggen M, Epple M (2020) Synthesis, structure, properties, and applications of bimetallic nanoparticles of noble metals. Adv Func Mater 30(21):1909260. https://doi.org/10.1002/adfm.201909260
Luby SP, Rahman M, Arnold BF, Unicomb L, Ashraf S, Winch PJ et al (2018) Effects of water quality, sanitation, handwashing, and nutritional interventions on diarrhoea and child growth in rural Bangladesh: a cluster randomised controlled trial. Lancet Global Health 6(3):e302–e315
Magana-Arachchi DN, Wanigatunge RP (2020) Ubiquitous waterborne pathogens. In: Waterborne pathogens, pp 15–42. Butterworth-Heinemann
Makvandi P, Wang C, Zare EN, Borzacchiello A, Niu L, Tay FR (2020) Metal-based nanomaterials in biomedical applications: antimicrobial activity and cytotoxicity aspects. Adv Func Mater 30(22):1910021. https://doi.org/10.1002/adfm.201910021
Manetu WM, Karanja AM (2021) Waterborne disease risk factors and intervention practices: a review. Oalib 08(05):1–11. https://doi.org/10.4236/oalib.1107401
McKee AM, Cruz MA (2021) Microbial and viral indicators of pathogens and human health risks from recreational exposure to waters impaired by fecal contamination. J Sustain Water Built Environ 7(2):03121001
Mohammed Sadiq I, Chandrasekaran N, Mukherjee A (2010) Studies on effect of TiO 2 nanoparticles on growth and membrane permeability of Escherichia coli , Pseudomonas aeruginosa , and Bacillus subtilis . Curr Nanosci 6(4):381–387. https://doi.org/10.2174/157341310791658973
Article ADS Google Scholar
Montgomery MA, Elimelech M (2007) Water and sanitation in developing countries: including health in the equation. Environ Sci Technol 41(1):17–24. https://doi.org/10.1021/es072435t
Article ADS PubMed Google Scholar
Montufar Salcedo C (2018) Modification of the treatment protocol as a strategy in the control of the cholera epidemic in Haiti 2016–2017. Med Case Rep Rev 1(3):1–3
Mulugeta S, Helmreich B, Drewes JE, Nigussie A (2020) Consequences of fluctuating depth of filter media on coliform removal performance and effluent reuse opportunities of a bio-sand filter in municipal wastewater treatment. J Environ Chem Eng 8(5):104135. https://doi.org/10.1016/j.jece.2020.104135
Murei A, Mogane B, Mothiba DP, Mochware OTW, Sekgobela JM, Mudau M et al (2022) Barriers to water and sanitation safety plans in rural areas of South Africa—a case study in the Vhembe District, Limpopo Province. Water 14(8):1244
Mutuma BK, Shao GN, Kim WD, Kim HT (2015) Sol–gel synthesis of mesoporous anatase–brookite and anatase–brookite–rutile TiO 2 nanoparticles and their photocatalytic properties. J Colloid Interface Sci 442:1–7
Mwambete KD, Tairo VP (2018) Bacteriological quality of household drinking water and water disinfection practices in Kinondoni Municipality, Tanzania. Int J Health Sci 1:10
Nigay P-M, Salifu AA, Obayemi JD, White CE, Nzihou A, Soboyejo WO (2019) Ceramic water filters for the removal of bacterial, chemical, and viral contaminants. J Environ Eng 145(10):04019066. https://doi.org/10.1061/(ASCE)EE.1943-7870.0001579
Norton DM, Rahman M, Shane AL, Hossain Z, Kulick RM, Bhuiyan MI, Wahed MA, Yunus M, Islam MS, Breiman RF, Henderson A, Keswick BH, Luby SP (2009) Flocculant-disinfectant point-of-use water treatment for reducing arsenic exposure in rural Bangladesh. Int J Environ Health Res 19(1):17–29. https://doi.org/10.1080/09603120802272219
Obafunmi T, Ocheme J, Gajere B (2020) Oligodynamic effect of precious metals on skin bacteria. Fudma J Sci 4(3):601–608. https://doi.org/10.33003/fjs-2020-0403-334
Okoh EO, Miner CA, Envuladu EA, Mohammed A, Ugochi J (2020) Effect of household water treatment on microbiological quality of drinking water in rural communities of Plateau State, Nigeria: a comparative study of two treatment modalities
Paliwal I (2021) Detection of Trichomonas vaginalis , Giardia and Cryptosporidium spp. in remote indigenous communities in Canada using a point-of-care device
Parham S, Wicaksono DHB, Bagherbaigi S, Lee SL, Nur H (2016) Antimicrobial treatment of different metal oxide nanoparticles: a critical review. J Chin Chem Soc 63(4):385–393. https://doi.org/10.1002/jccs.201500446
Peterson KM, Diedrich DE, Lavigne DJ (2008) Strategies for combating waterborne diarrheal diseases in the developing world, 39.
Praveena SM, Aris AZ (2015) Application of low-cost materials coated with silver nanoparticle as water filter in Escherichia coli removal. Water Qual Expo Health 7(4):617–625. https://doi.org/10.1007/s12403-015-0167-5
Quang DV, Sarawade PB, Hilonga A, Kim J-K, Shim YH, Shao GN, Kim HT (2012) Synthesis of silver nanoparticles within the pores of functionalized-free silica beads: the effect of pore size and porous structure. Mater Lett 68:350–353. https://doi.org/10.1016/j.matlet.2011.10.073
Raghunath A, Perumal E (2017) Metal oxide nanoparticles as antimicrobial agents: a promise for the future. Int J Antimicrob Agents 49(2):137–152. https://doi.org/10.1016/j.ijantimicag.2016.11.011
Rayner J, Luo X, Schubert J, Lennon P, Jellison K, Lantagne D (2017) The effects of input materials on ceramic water filter efficacy for household drinking water treatment. Water Supply 17(3):859–869. https://doi.org/10.2166/ws.2016.176
Reece SM, Sinha A, Grieshop AP (2017) Primary and photochemically aged aerosol emissions from biomass cookstoves: chemical and physical characterization. Environ Sci Technol 51(16):9379–9390.
Rehman AU, Nazir S, Irshad R, Tahir K, ur Rehman K, Islam RU, Wahab Z (2021) Toxicity of heavy metals in plants and animals and their uptake by magnetic iron oxide nanoparticles. J Mol Liq 321:114455
Sawai J, Himizu K, Yamamoto O (2005) Kinetics of bacterial death by heated dolomite powder slurry. Soil Biol Biochem 37(8):1484–1489. https://doi.org/10.1016/j.soilbio.2005.01.011
Shailemo DHP, Kwaambwa HM, Kandawa-Schulz M, Msagati TAM (2016) Antibacterial activity of Moringa ovalifolia and Moringa oleifera methanol, N-hexane and water seeds and bark extracts against pathogens that are implicated in water borne diseases. Green Sustain Chem 06(02):71–77. https://doi.org/10.4236/gsc.2016.62006
Shao GN, Engole M, Imran SM, Jeon SJ, Kim HT (2015) Sol–gel synthesis of photoactive kaolinite-titania: effect of the preparation method and their photocatalytic properties. Appl Surf Sci 331:98–107. https://doi.org/10.1016/j.apsusc.2014.12.199
Shao GN, Imran SM, Jeon SJ, Engole M, Abbas N, Salman Haider M, Kang SJ, Kim HT (2014) Sol–gel synthesis of photoactive zirconia–titania from metal salts and investigation of their photocatalytic properties in the photodegradation of methylene blue. Powder Technol 258:99–109. https://doi.org/10.1016/j.powtec.2014.03.024
Sobsey MD, Brown J (2012) Boiling as household water treatment in Cambodia: a longitudinal study of boiling practice and microbiological effectiveness. Am J Trop Med Hyg 87(3):394–398. https://doi.org/10.4269/ajtmh.2012.11-0715
Syafrudin M, Kristanti RA, Yuniarto A, Hadibarata T, Rhee J, Al-onazi WA, Algarni TS, Almarri AH, Al-Mohaimeed AM (2021) Pesticides in drinking water—a review. Int J Environ Res Public Health 18(2):468. https://doi.org/10.3390/ijerph18020468
Thill A, Zeyons O, Spalla O, Chauvat F, Rose J, Auffan M, Flank AM (2006) Cytotoxicity of CeO 2 nanoparticles for Escherichia coli . physico-chemical insight of the cytotoxicity mechanism. Environ Sci Technol 40(19):6151–6156. https://doi.org/10.1021/es060999b
Tsao NH, Malatesta KA, Anuku NE, Soboyejo WO (2015) Virus filtration in porous iron (III) oxide doped ceramic water filters. Adv Mater Res 1132:284–294. https://doi.org/10.4028/www.scientific.net/AMR.1132.284
Ubomba-Jaswa E, Navntoft C, Polo-López MI, Fernandez-Ibáñez P, McGuigan KG (2009) Solar disinfection of drinking water (SODIS): an investigation of the effect of UV-A dose on inactivation efficiency. Photochem Photobiol Sci 8(5):587. https://doi.org/10.1039/b816593a
UN (2019) The United Nations world water development report 2019: leaving no one behind
Vargas-Reus MA, Memarzadeh K, Huang J, Ren GG, Allaker RP (2012) Antimicrobial activity of nanoparticulate metal oxides against peri-implantitis pathogens. Int J Antimicrob Agents 40(2):135–139. https://doi.org/10.1016/j.ijantimicag.2012.04.012
Vega-Jiménez AL, Vázquez-Olmos AR, Acosta-Gío E, Álvarez-Pérez MA (2019) In vitro antimicrobial activity evaluation of metal oxide nanoparticles. Nanoemulsions Prop Fabr Appl 78812(2):1–18
Verma S, Daverey A, Sharma A (2017) Slow sand filtration for water and wastewater treatment—a review. Environ Technol Rev 6(1):47–58
Waddington H, Snilstveit B (2009) Effectiveness and sustainability of water, sanitation, and hygiene interventions in combating diarrhoea. J Dev Eff 1(3):295–335. https://doi.org/10.1080/19439340903141175
Wang Z, Lee Y-H, Wu B, Horst A, Kang Y, Tang YJ, Chen D-R (2010) Anti-microbial activities of aerosolized transition metal oxide nanoparticles. Chemosphere 80(5):525–529. https://doi.org/10.1016/j.chemosphere.2010.04.047
Wen X, Chen F, Lin Y, Zhu H, Yuan F, Kuang D et al (2020) Microbial indicators and their use for monitoring drinking water quality—a review. Sustainability 12(6):2249
Weststrate J, Dijkstra G, Eshuis J, Gianoli A, Rusca M (2019) The sustainable development goal on water and sanitation: learning from the millennium development goals. Soc Indic Res 143:795–810
World Health Organization (2006) Guidelines for drinking-water quality: First addendum to the third edition, volume 1: recommendations
World Health Organization (2022) Guidelines for drinking‑water quality: Fourth edition incorporating the first and second addenda (4th ed + 1st add + 2nd add). World Health Organization. https://apps.who.int/iris/handle/10665/352532
World Health Organization (2020) State of the world’s sanitation: an urgent call to transform sanitation for better health, environments, economies and societies
Yekta R, Vahid-Dastjerdi L, Norouzbeigi S, Mortazavian AM (2021) Food products as potential carriers of SARS-CoV-2. Food Control 123:107754
Download references
Acknowledgements
We acknowledge Mkwawa University College of Education (MUCE) for material support
Author information
Authors and affiliations.
Department of Chemistry, Mkwawa University College of Education, University of Dar es Salaam, P.O. Box 2513, Iringa, Tanzania
Godfrey Michael Shayo, Elianaso Elimbinzi, Godlisten N. Shao & Christina Fabian
You can also search for this author in PubMed Google Scholar
Contributions
GMS participated in designing, writing, and submitting the manuscript. GNS originated the water purification system idea and conducted research relating to chemical contaminants in water and final approval of the version to be submitted. CFP involved in organization of the manuscript, editing of the manuscript, and revision of the manuscript critically for important intellectual content. EE was a major contributor to the manuscript's writing and interpretation of the relevant literature. All authors have read and approved the manuscript.
Corresponding author
Correspondence to Godfrey Michael Shayo .
Ethics declarations
Ethics approval and consent to participate, consent for publications, competing interests.
The authors declare that they have no competing interests.
Additional information
Publisher's note.
Springer Nature remains neutral with regard to jurisdictional claims in published maps and institutional affiliations.
Rights and permissions
Open Access This article is licensed under a Creative Commons Attribution 4.0 International License, which permits use, sharing, adaptation, distribution and reproduction in any medium or format, as long as you give appropriate credit to the original author(s) and the source, provide a link to the Creative Commons licence, and indicate if changes were made. The images or other third party material in this article are included in the article's Creative Commons licence, unless indicated otherwise in a credit line to the material. If material is not included in the article's Creative Commons licence and your intended use is not permitted by statutory regulation or exceeds the permitted use, you will need to obtain permission directly from the copyright holder. To view a copy of this licence, visit http://creativecommons.org/licenses/by/4.0/ .
Reprints and permissions
About this article
Cite this article.
Shayo, G.M., Elimbinzi, E., Shao, G.N. et al. Severity of waterborne diseases in developing countries and the effectiveness of ceramic filters for improving water quality. Bull Natl Res Cent 47 , 113 (2023). https://doi.org/10.1186/s42269-023-01088-9
Download citation
Received : 05 May 2023
Accepted : 17 July 2023
Published : 24 July 2023
DOI : https://doi.org/10.1186/s42269-023-01088-9
Share this article
Anyone you share the following link with will be able to read this content:
Sorry, a shareable link is not currently available for this article.
Provided by the Springer Nature SharedIt content-sharing initiative
- Waterborne diseases
- Developing countries
- Drinking water
- Water contaminants
- Water-filtration strategies
- Water quality
Thank you for visiting nature.com. You are using a browser version with limited support for CSS. To obtain the best experience, we recommend you use a more up to date browser (or turn off compatibility mode in Internet Explorer). In the meantime, to ensure continued support, we are displaying the site without styles and JavaScript.
- View all journals
- Explore content
- About the journal
- Publish with us
- Sign up for alerts
- Published: 20 April 2020
Cascading risks of waterborne diseases from climate change
- Jan C. Semenza ORCID: orcid.org/0000-0002-4625-874X 1
Nature Immunology volume 21 , pages 484–487 ( 2020 ) Cite this article
3479 Accesses
56 Citations
15 Altmetric
Metrics details
- Infectious diseases
- Scientific community
Climate change can trigger a sequence of events of significant magnitude with consequences for waterborne diseases. Heavy rainfall, flooding and hot weather are associated with waterborne diseases, but early warning systems could intercept these cascading risks.
This is a preview of subscription content, access via your institution
Relevant articles
Open Access articles citing this article.
Perspectives on climate change and infectious disease outbreaks: is the evidence there?
- Gina E. C. Charnley
- & Ilan Kelman
npj Climate Action Open Access 20 July 2024
Climate Change and Cascading Risks from Infectious Disease
Jan C. Semenza
- , Joacim Rocklöv
- & Kristie L. Ebi
Infectious Diseases and Therapy Open Access 19 May 2022
Access options
Access Nature and 54 other Nature Portfolio journals
Get Nature+, our best-value online-access subscription
24,99 € / 30 days
cancel any time
Subscribe to this journal
Receive 12 print issues and online access
195,33 € per year
only 16,28 € per issue
Buy this article
- Purchase on SpringerLink
- Instant access to full article PDF
Prices may be subject to local taxes which are calculated during checkout

Semenza, J. C. et al. Transbound. Emerg. Dis. 66 , 1855–1863 (2019).
Article Google Scholar
Semenza, J. C. & Menne, B. Lancet Infect. Dis. 9 , 365–375 (2009).
IPCC. Special Report on Global Warming of 1 .5 °C (eds Masson-Delmotte, V. P. et al.) (WMO, 2018).
Suk, J. E., Vaughan, E. C., Cook, R. G. & Semenza, J. C. Eur. J. Public Health https://doi.org/10.1093/eurpub/ckz111 (2019).
Guzman Herrador, B. R. et al. Environ. Health 14 , 29 (2015).
Levy, K., Woster, A. P., Goldstein, R. S. & Carlton, E. J. Environ. Sci. Technol. 50 , 4905–4922 (2016).
Article CAS Google Scholar
Lo Iacono, G. et al. PLoS Negl. Trop. Dis. 11 , e0005659 (2017).
Semenza, J. C. et al. Crit. Rev. Environ. Sci. Technol. 42 , 857–890 (2012).
Curriero, F. C., Patz, J. A., Rose, J. B. & Lele, S. Am. J. Public Health 91 , 1194–1199 (2001).
Guzman Herrador, B. R. et al. J. Water Health 14 , 1019–1027 (2016).
Thomas, K. M. et al. Int. J. Environ. Health Res. 16 , 167–180 (2006).
Nichols, G., Lane, C., Asgari, N., Verlander, N. Q. & Charlett, A. J. Water Health 7 , 1–8 (2009).
Semenza, J. C. & Nichols, G. Euro Surveill. 12 , E13-4 (2007).
Sterk, A., Schijven, J., de Roda Husman, A. M. & de Nijs, T. Water Res. 95 , 90–102 (2016).
Adkins, H. J. et al. J. Clin. Microbiol. 25 , 1143–1147 (1987).
Dewan, A. M., Corner, R., Hashizume, M. & Ongee, E. T. PLoS Negl. Trop. Dis. 7 , e1998 (2013).
Desai, S. et al. Clin. Infect. Dis. 48 , 691–697 (2009).
Kelley, C. P., Mohtadi, S., Cane, M. A., Seager, R. & Kushnir, Y. Proc. Natl Acad. Sci. USA 112 , 3241–3246 (2015).
Singh, R. B. et al. Environ. Health Perspect. 109 , 155–159 (2001).
Effler, E. et al. Emerg. Infect. Dis. 7 , 812–819 (2001).
Schijven, J. et al. Risk Anal. 33 , 2154–2167 (2013).
Shapiro, R. S. & Cowen, L. E. MBio 3 , e00238–12 (2012).
Cherrie, M. P. C. et al. BMC Public Health 18 , 1067 (2018).
Lake, I. R. Environ. Health 16 (Suppl. 1), 117 (2017).
Yun, J. et al. Sci. Rep. 6 , 28442 (2016).
Hume, R., Berndt, K. D., Normark, S. J. & Rhen, M. Cell 90 , 55–64 (1997).
Djennad, A. et al. BMC Infect. Dis. 19 , 255 (2019).
Lake, I. R. et al. Euro Surveill. 24 , 180028 (2019).
Baker-Austin, C. et al. Emerg. Infect. Dis. 22 , 1216–1220 (2016).
Semenza, J. C. et al. Environ. Health Perspect. 125 , 107004 (2017).
Damania, R. et al. Uncharted Waters : The New Economics of Water Scarcity and Variability (World Bank, 2017).
European Centre for Disease Prevention and Control. Vibrio map viewer. E3 Geoportal https://e3geoportal.ecdc.europa.eu/SitePages/Vibrio%20Map%20Viewer.aspx (2020).
Download references
Acknowledgements
I would like to thank J. Takkinen and M. Catchpole at the European Centre for Disease Prevention and Control for critical feedback on the manuscript. The views and opinions expressed herein are the author’s own and do not necessarily state or reflect those of the ECDC. The ECDC is not responsible for the data and information collation and analysis and cannot be held liable for conclusions or opinions drawn.
Author information
Authors and affiliations.
European Centre for Disease Prevention and Control (ECDC), Scientific Assessment Section https://www.ecdc.europa.eu/en
You can also search for this author in PubMed Google Scholar
Corresponding author
Correspondence to Jan C. Semenza .
Ethics declarations
Competing interests.
The author declares no competing interests.
Rights and permissions
Reprints and permissions
About this article
Cite this article.
Semenza, J.C. Cascading risks of waterborne diseases from climate change. Nat Immunol 21 , 484–487 (2020). https://doi.org/10.1038/s41590-020-0631-7
Download citation
Published : 20 April 2020
Issue Date : May 2020
DOI : https://doi.org/10.1038/s41590-020-0631-7
Share this article
Anyone you share the following link with will be able to read this content:
Sorry, a shareable link is not currently available for this article.
Provided by the Springer Nature SharedIt content-sharing initiative
Quick links
- Explore articles by subject
- Guide to authors
- Editorial policies
Sign up for the Nature Briefing newsletter — what matters in science, free to your inbox daily.

An official website of the United States government
Official websites use .gov A .gov website belongs to an official government organization in the United States.
Secure .gov websites use HTTPS A lock ( Lock Locked padlock icon ) or https:// means you've safely connected to the .gov website. Share sensitive information only on official, secure websites.
- Publications
- Account settings
- Advanced Search
- Journal List

Ubiquitous waterborne pathogens
Dn magana-arachchi, rp wanigatunge.
- Author information
- Article notes
- Copyright and License information
Issue date 2020.
Since January 2020 Elsevier has created a COVID-19 resource centre with free information in English and Mandarin on the novel coronavirus COVID-19. The COVID-19 resource centre is hosted on Elsevier Connect, the company's public news and information website. Elsevier hereby grants permission to make all its COVID-19-related research that is available on the COVID-19 resource centre - including this research content - immediately available in PubMed Central and other publicly funded repositories, such as the WHO COVID database with rights for unrestricted research re-use and analyses in any form or by any means with acknowledgement of the original source. These permissions are granted for free by Elsevier for as long as the COVID-19 resource centre remains active.
Water is one of the most important substances on earth and without it life cannot exist. However, poor water quality in many parts of the world has increased the number of water-related diseases, making it the leading cause of disease and death globally for both young and old. Waterborne pathogens cause diseases in humans through two major exposure pathways: drinking water and recreational waters. This chapter on waterborne pathogens will be starting with an introduction, followed by descriptions on classical waterborne pathogens; bacteria, viruses, protozoans, and helminths placing emphasis on the World Health Organization guidelines. Further to conventional waterborne pathogens, fresh organisms and new strains from already known pathogens are being identified and that present important additional challenges to both the water and public health sectors. Hence later part of the chapter focuses on the potential waterborne pathogens and will conclude with a summary of the content.
Keywords: Bacteria, Helminths, Protozoans, Viruses, Waterborne diseases, Waterborne pathogens
1. Introduction
In July 2010, the United Nations General Assembly (UNGA) univocally recognized the human right to water and sanitation and acknowledged that clean drinking water and sanitation are essential to the realization of all human rights ( UNGA, 2010 ). However, due to inadequacy, unsafe, inaccessibility, and unaffordability of water, most of the people globally are deprived of this universal right. According to the Joint Monitoring Program (JMP) report, some 3 in 10 people worldwide, or 2.1 billion, lack access to safe, readily available water at home and 6 in 10, or 4.5 billion, lack safely managed sanitation ( WHO and UNICEF, 2017 ). Due to the global efforts, billions of people have gained access to basic drinking water and sanitation services since 2000, but people in many countries are still lacking clean water and proper sanitation in their homes, healthcare facilities, and schools. Hence health of all these people is at a risk, affecting mainly the infants and young children. Water, sanitation, and hygiene were responsible for 829,000 deaths from diarrheal disease in 2016. It is estimated that every year, 361,000 children under 5 years of age die because of diarrhea. In addition, poor sanitation and contaminated water are also linked to transmission of waterborne diseases such as cholera, dysentery, hepatitis A, and typhoid ( WHO and UNICEF, 2017 ).
In September 2015, Member States of the United Nations adopted the 2030 Agenda for Sustainable Development ( UNSD, 2015 ) and Goal 6 of Sustainable Development Goals is to “ Ensure availability and sustainable management of water and sanitation for all .” Targets were set by considering the freshwater cycle as a whole. Member States try to achieve these targets by improving the standard of water, sanitation, and hygiene (WASH) services; increasing treatment, recycling, and reuse of wastewater; improving efficiency and ensuring sustainable withdrawals; and protecting water-related ecosystems as part of an integrated approach to water resources management. They also address the means of implementation for achieving these development outcomes ( WHO and UNICEF, 2017 ).
A pathogen means an agent that causes disease to a host, and waterborne pathogens are the causative agents (usually living organisms) for diseases that are being transmitted through water. Water pollution can occur due to chemical and/or biological contaminants. These waterborne pathogens thrive in polluted waters, especially contaminated with human feces or/and urine. People could get exposed to these microorganisms while drinking water, by eating food prepared with contaminated water, bathing, during recreational activities, or even sometimes in healthcare facilities during dialysis. This exposure could be limited to an individual or it can be a community outbreak. The morbidity and mortality caused by contaminated water are enormous and it could only be controlled by providing microbiologically safe and toxin-free water for drinking, cooking, and other recreational activities.
Surface waters in most countries are polluted with pathogens and this is widely recorded in the developing world. Consumption of these waters leads to waterborne disease outbreaks (WBDOs) ( Patel et al., 2016 ). A recent study from China has shown that potentially pathogenic bacteria were ubiquitous across all of the 16 urban sampled surface waters, and Proteobacteria and Bacteroidetes were the most commonly detected phyla accounting for 21.9%–78.5% and 19.1%–74.7% of sequences, respectively ( Jin et al., 2018 ). Intermittent water supply (IWS) is being practiced throughout low- to middle-income countries. A study was conducted by Bivins et al. (2017) with existing data using reference pathogens Campylobacter , Cryptosporidium , and rotavirus (RV) as conservative risk proxies for infections via bacteria, protozoa, and viruses, respectively. Their findings indicated that the median daily risk of infection is 1 in 23,500 for Campylobacter , 1 in 5,050,000 for Cryptosporidium , and 1 in 118,000 for RV. Based on these risks, IWS may account for 17.2 million infections causing 4.52 million cases of diarrhea, 109,000 diarrheal disability-adjusted life years (DALYs), and 1560 deaths each year. The WHO health-based normative guideline for drinking water is 10 −6 DALYs per person per year and it is likely that the value of diarrheal disease associated with IWS will be surpassing the WHO value.
When ensuring clean water for drinking and other activities, proper management guidelines are needed to be followed. Preparation of these guidelines is not an easy task and it needs thorough understanding about the pathogenic nature of the organisms considering their shape, size, composition, and structure, their survival and behavior, and how they transmit in different waterbodies. Many countries use indicator organisms to assess the microbiological quality of drinking water. Most widely used bacteria are the enteric bacterial coliforms belonging to the family Enterobacteriaceae. In addition to being able to identify the microbial contamination of drinking water with human waste, these organisms are capable of identifying the fecal contaminations in waters used for recreational activities as well as in shellfish production. The presence of these pathogenic organisms in waterbodies is being monitored regularly in most developed countries as new tools are available to them due to the advances made in medical and scientific research. Modern technologies have also been incorporated into the water treatment plants for the effective removal or deactivation of these waterborne pathogens, thereby minimizing the outbreaks and the risk due to exposure.
In this chapter we will be focusing on the ubiquitous waterborne pathogens which cause deadly diseases and outbreaks affecting young and old globally. Etiological agents for substantial amount of waterborne diseases are “classical” waterborne pathogens. However, fresh organisms and new strains from already known pathogens are being identified and that present important additional challenges to both the water and public health sectors. Hence we will outline the potential waterborne pathogens including Helicobacter pylori , Tsukamurella , Cystoisospora belli , and Microsporidia and also Bacillus species and toxic cyanobacteria that needed to be paid attention to supply clean water, prevent mishaps, and protect and improve public health. Listed pathogens for this chapter were selected from the WHO Guidelines for drinking water quality, fourth edition ( 2011 ) and from Global Waterborne Pathogen Project (GWPP) ( Rusinol and Girones, 2017 ). Readers could gain extra knowledge on these organisms by referring to the original articles which are being included in the references. Furthermore, descriptive diagrams of these organisms can be found in the book chapter by Bridle (2013) .
2. Waterborne pathogens
This first section is based on ubiquitous waterborne pathogens including bacteria, viruses, protozoa, and helminths, which will be discussed in chronological order, and the causative diseases and mode of transmission are summarized schematically in Fig. 2.1 for the readers' benefit.
Figure 2.1.
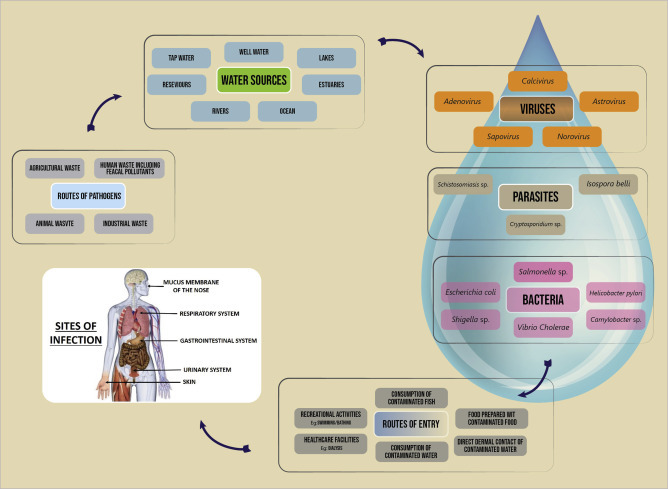
Generalized overview of ubiquitous waterborne pathogens, route of transmission, and sites of infection.
2.1. Waterborne bacteria
Bacterial pathogens are classical etiological agents of waterborne diseases globally. These organisms can occur ubiquitously in many aquatic habitats and humid soils. They are an important part of the biocenosis in various substrates or water systems, especially in their preferred habitats, the biofilms. According to WHO, from the mortality of water-associated diseases, more than 50% are due to microbial intestinal infections. There are limitations in many of the established methods used in water quality assessments, and new approaches to health-related monitoring are being introduced by WHO that can overcome many of the weaknesses in current methods and provide additional tools for reducing disease risks ( WHO, 2003 ).
2.1.1. The genus Vibrio
Vibrios are small, curved-shaped or rods, facultative anaerobes with a single polar flagellum, belonging to family Vibrionaceae of order Vibrionales that are non–spore-forming and Gram-negative with a size of approximately 1.5–3.0 μm × 0.5 μm. Cells of Vibrio cholerae, Vibrio parahaemolyticus , and Vibrio vulnificus possess pili (fimbriae) structures comprising of protein TcpA. This TcpA formation is co-regulated with cholera toxin expression and is a key determinant of in vivo colonization.
These are primarily aquatic bacteria, common in marine and estuarine environments, living free or on the surfaces and in the intestinal contents of marine animals. Around 12 Vibrio species can infect humans. V. cholerae is, by far, the most important among them. Vibrio fluvialis , Grimontia hollisae (formerly Vibrio hollisae ), and Vibrio mimicus can cause diarrhea or infections of the gastrointestinal tract. Vibrio furnissii has been isolated from a few individuals with diarrhea, but there is no evidence that it can actually cause this pathology.
Cholera is a well-known disease since 19 th century and it is topping the list of microbial waterborne diseases. V. cholerae is a very diverse bacterial species. It has more than 200 serovarieties, characterized by the structure of the lipopolysaccharide (O antigens). Only serovarieties O1 and O139 are involved in true cholera ( Weintraub, 2003 ). Some other serovarieties can cause gastroenteritis. The serovariety O1 is subdivided into classical and El Tor biotypes based on their biochemical properties and phage susceptibilities. Only toxigenic strains have the CTXΦ segment (7–9.7 kb) of the chromosome and this carries at least six genes which have the potential to encode cholera toxin. During chromosome replication, the CTXΦ fragment is able to make an autonomous copy creating an independent plasmid. The plasmid produces virus-like particles, the CTXΦ bacteriophages, which in turn infect nontoxigenic strains ( Cabral, 2010 ). Epidemic and pandemic strains of V. cholerae contain another chromosomal segment designated as Vibrio Pathogenicity Island (VPI). VPI is 39.5 kb in size and contains two ToxR-regulated genes: a regulator of virulence genes (ToxT) and a gene cluster containing colonization factors, including the toxin co-regulated pili. Pathogen can be transmitted by the contaminated water or food via the fecal–oral route. V. cholerae O1 or O139 strains are common in estuaries being isolated from estuarine animals, such as birds, frogs, fishes, and shellfish, and are able to survive and multiply on the surface of phytoplankton and zooplankton cells.
2.1.2. The genus Salmonella
Salmonellae are rod-shaped, motile by peritrichous flagella, belonging to family Enterobacteriaceae of order Enterobacteriales that are non–spore-forming, Gram-negative bacteria with a size of a rod being 0.7–1.5 μm by 2.2–5.0 μm producing colonies approximately 2–4 mm in diameter. Salmonellae have several endotoxins: antigens O, H, and Vi.
Salmonella enterica subsp. enteric serovar Enteritidis is the most frequently isolated serovar from humans all over the world. However, other serovars can be predominant and each outbreak had been associated with a different serotype: Mbandaka, Livingstone, and Typhi Vi+. The major habitat of Salmonella is the intestinal tract of humans and animals and is frequently found in environmental samples because they are excreted by humans as well as animals. Municipal sewage, agricultural waste, and storm water runoff are the main sources of these pathogens in natural waters and they do not multiply much in natural environments but can survive several weeks in water and soil when environmental factors are favorable. Paratyphi or non-Typhi serovars of Salmonellae are more common in the environment. From environmental sources, 73% of the isolates were from tap water in which commonly observed organisms being serovars: Corvallis, Enteritidis, and Anatum ( Aissa et al., 2007 ). A study reported a total of 19 Salmonella serotypes in a comparative study carried out in rivers Aliakmon and Axios, in northern Greece ( Arvanitidou et al., 2005 ).
2.1.3. The genus Shigella
Shigellae are rod-shaped and nonmotile, belonging to family Enterobacteriaceae of order Enterobacteriales that are non–spore-forming, Gram-negative with a size of a cell being 0.4–0.6 μm by 1.0–3.0 μm long. There are four serogroups in Shigella : Shigella dysenteriae (serogroup A) with 1–15 serotypes, Shigella flexneri (serogroup B) serotypes 1–8 with 9 subtypes, Shigella boydii (serogroup C) with serotypes 1–19, and Shigella sonnei (serogroup D) with one serotype. The four serogroups differ in their epidemiology and outbreaks have been attributed to the community water supplies which were not properly chlorinated.
Shigella has a complex antigenic pattern and the serogrouping is based on their somatic O antigens. Shigella emerged from Escherichia coli during evolution. The acquisition and evolution of the pathogenicity island, which encodes all of the genes required for cell invasion and phagolysosomal lysis, permitted a major alteration in pathogenesis. Shigella is the causative agent for the disease shigellosis or bacillary dysentery, naturally spread by fecal-contaminated drinking water or food or by direct contact with an infected person and considered as a disease affecting the under developed displaced people who are lacking the basic hygienic facilities.
2.1.4. The genus Escherichia
Bacteria in genus Escherichia are rod-shaped, non–spore-forming, Gram-negative bacteria belonging to family Enterobacteriaceae of order Enterobacteriales. Commonly found E. coli have a size of 2.0–0.5 μm in diameter. E. coli is a natural and essential part of the bacterial flora in the gut of humans and animals. Most E. coli strains inhabiting colon are nonpathogenic, but certain serotypes have a role in intestinal and extraintestinal diseases, such as urinary tract infections.
There are six different groups of E. coli strains isolated from intestinal diseases based on epidemiological evidence, phenotypic traits, clinical features of the disease, and specific virulence factors. Among them, enterotoxigenic E. coli O148, enterohemorrhagic E. coli O157, and enteroinvasive E. coli O124 serotypes are major disease-causing organisms and can be transmitted through contaminated water.
Enterotoxigenic E. coli (ETEC) serotypes can cause infantile gastroenteritis. Disease is caused due to consumption of ETEC-contaminated food or water and is characterized by profuse watery diarrhea continuing for several days leading to dehydration and malnutrition in young children. ETEC serotype 148 is one of the causative agents of “travelers' diarrhea” that affects individuals who are involved in global traveling.
Shiga toxin-producing E. coli O157:H7 is considered as food and waterborne pathogen that causes diarrhea, hemorrhagic colitis, and hemolytic uremic syndrome (HUS) in humans in both sporadic cases and outbreaks ( Wasey and Salen, 2019 ). The incubation period is 3–4 days, and the symptoms last for 7–10 days. HUS associated with Shiga toxin-producing E . coli O157:H7 cause acute renal failure mostly in children. These bacteria are naturally not a concern in treated drinking water, but outbreaks related to consumption of contaminated water or use of surface water for recreational activities have been documented ( Bruneau et al., 2004 ). Enterohemorrhagic E . coli have also been isolated from ponds, streams, wells, and water troughs, and they can survive for months in manure and water-trough sediments. Personal contacts are an important mode of transmission and disease spread through the oral–fecal route.
Enteroinvasive E. coli act as same as Shigella . They are capable of invading and multiplying in the intestinal epithelial cells of the distal large bowel in humans. The illness is characterized by abdominal cramps, diarrhea, vomiting, fever, chills, a generalized malaise, and the appearance of blood and mucus in the stools of infected individuals. E. coli O124 had been isolated from cases of gastroenteritis, enterocolitis, and dysentery. Food prepared by using water contaminated with human waste could cause the disease in humans.
2.1.5. The genus Burkholderia
Bacteria in the genus Burkholderia are straight or slightly curved, rod-shaped, non–spore-forming and Gram-negative, and motile due to a single or multiple polar flagella except in one species, belonging to family Burkholderiaceae of order Burkholderiales. The genus comprises of 60 species of obligatory aerobes that are ubiquitous in nature and are waterborne pathogens. Clinically relevant species include Burkholderia cepacia complex species, Burkholderia pseudomallei , Burkholderia mallei , Burkholderia gladioli . B . pseudomallei is with a diameter of 0.8 μm, and a length of 1.5 μm can be present in soil or water whether it is surface or ground. Melioidosis is a life-threatening disease caused by B. pseudomallei and is endemic to southeast Asians and to northern Australians and randomly affects people living close to the equator. It is more common during the monsoon season than in dry months and literature confirms that it became more prevalent after 2004 Tsunami ( Currie et al., 2008 ).
Melioidosis has been reported from Thailand and the disease is highly endemic to the northeast. Most infected community is agricultural farmers with repeated environmental exposure ( Limmathurotsakul et al., 2013 ). Water supply–related melioidosis has also been documented and the disease could be acute or chronic. Signs and symptoms may include pain in the chest, bones, or joints; cough; skin infections, lung nodules, and pneumonia, which is a life-threatening infection that is estimated to account for nearly 89,000 deaths per year worldwide ( Wiersinga et al., 2018 ). Melioidosis is an emerging disease in Sri Lanka ( Corea et al., 2012 ).
2.1.6. The genus Campylobacter
The bacteria in genus Campylobacter are Gram-negative, 0.5–8 μm long, and 0.2–0.5 μm wide with characteristically curved, spiral, or S-shaped cells belonging to the family Campylobacteraceae in order Campylobacterales. This genus consists of 29 species and 12 subspecies. The most important Campylobacter species in human gastroenteritis is Campylobacter jejuni followed by Campylobacter coli , Campylobacter lari , and Campylobacter fetus . Campylobacter enteritis was the causative agent for 8.5% of the total burden of diarrheal disease, standing fourth after RV, cryptosporidiosis, and E. coli diarrhea (combined enterotoxigenic and enteropathogenic E. coli infections) ( Murray et al., 2012 ).
Disparities have been observed between developed and developing countries in the epidemiology and demography of Campylobacter infections. In developing countries, symptomatic disease is most commonly seen only during the first 2 years of life, and symptomatic illness in adults is scarce because of the endemic nature. In developed world, the most common symptoms include an acute, self-limiting gastroenteritis, with an incubation period of 2–5 days, whereas in developing nations, watery diarrhea is mostly observed. This is considered also as a zoonotic disease and wide variety of animals, especially poultry, wild birds, cattle, and sheep carry high numbers of C. jejuni and C. coli as commensals in their intestines. Fecal contamination of food, recreational water, and drinking water contributes to human infections and the fecal material of infected persons spread the organisms back to environment through sewage plants and toilets.
2.1.7. The genus Francisella
The genus Francisella is 0.7–1.7 μm in size, nonmotile, Gram-negative, strictly aerobic, and facultative intracellular coccobacilli species. The type species of the genus is the Francisella tularensis , which contains four subspecies, i.e., tularensis , holarctica , mediasiatica , and novicida . F. tularensis is a highly infectious bacterium causing disease in mammals including humans and a potential bioterror weapon ( Colquhoun et al., 2014 ). Tularemia is a zoonotic infection caused by F. tularensis mainly transmitted to humans through arthropod bites, direct contact with infected animals, and inhalation or ingestion of contaminated water. The organism can persist in water or mud at least for 1 year and that indicates the environment may be important reservoirs for this pathogen. Recent and historical outbreaks indicate that environmental exposure to the organism is a significant source of morbidity.
2.1.8. The genus Legionella
Legionellae are rod-shaped, Gram-negative bacteria being the only genus in family Legionellaceae. The genus includes 52 validated species with 71 serotypes out of which 24 Legionella species are described as occasional human pathogens. Legionella pneumophila cause Legionnaires' disease, which is a sever type of pneumonia occurring worldwide. The transmission can occur via inhalation of contaminated aerosols generated by cooling towers, bath tubs, whirlpools, ornamental fountains, and showers. In nature, Legionella live in freshwater and rarely cause illness. Outbreaks of Legionnaires' disease are often associated with man-made water settings, with large or complex water systems. Most outbreaks have been due to L. pneumophila , serogroup 1, and although this may be due to increased virulence, it may simply reflect the greater prevalence of this particular organism ( Yu et al., 2002 ). Biofilms and free-living amebae are considered to serve as main environmental reservoirs for L. pneumophila and represent a potential source of drinking water contamination, resulting in a potential health risk for humans.
2.1.9. Mycobacterium avium complex
The genus Mycobacterium belongs to family Mycobacteriaceae of order Actinomycetales. The Mycobacterium avium complex (MAC) consists of 28 serovars of two distinct species: Mycobacterium avium and Mycobacterium intracellulare. MAC has been recovered from drinking water systems both before and after treatment, hot water heaters, freshwater, brackish, sea water, and wastewater, occasionally being high in numbers, and the infection to humans occurs through the inhalation of aerosolized droplets containing M. avium cells.
The organisms of MAC have the ability to survive and grow under diverse and extreme conditions. Hence mycobacteria are highly resistant to chlorine and other chemical disinfectants as such standard drinking water treatments will not completely eliminate MAC organisms but can minimize the risk. The symptoms encountered with MAC infections result from colonization of either the respiratory or the gastrointestinal tract, with possible dissemination to other locations in the body.
2.2. Waterborne viruses
Diarrheal disease and WBDOs from drinking, recreational, and groundwaters are often caused by waterborne viruses, which tend to be more persistent in the environment than bacteria ( Gibson, 2014 ). WHO has classified adenovirus (AdV), astrovirus (AstV), hepatitis A and E viruses, RV, norovirus, and other caliciviruses and enteroviruses, including coxsackieviruses and polioviruses as water-transmitted viral pathogens having a moderate to high health significance ( WHO, 2011 ). Also, polyomaviruses and cytomegalovirus that are excreted through urine can potentially be spread through water. Influenza and coronaviruses have been proposed as organisms that can be transmitted through drinking water, but evidences are lacking. These viruses are mostly associated with gastroenteritis, which can cause diarrhea as well as other symptoms including abdominal cramping, vomiting, and fever. Some of these same viruses could also cause more severe illnesses including encephalitis, meningitis, myocarditis (enteroviruses), cancer (polyomavirus), and hepatitis (hepatitis A and E viruses) ( WHO, 2011 ).
2.2.1. Adenoviruses
AdVs, belonging to the family Adenoviridae, genus Mastadenovirus , have over 51 serotypes differentiated to six subgroups (A to F), which are the causative agents of many human diseases. They are 80–90 nm in size containing double-stranded linear DNA and a nonenveloped icosahedral shell that has slender projections from each of its 12 vertices. They can infect many organs in the body including the eye, upper respiratory tract, lower respiratory tract, gastrointestinal tract (gastroenteritis and intussusception), urinary bladder, central nervous system, and genitalia. The enteric adenoviruses types 31, 40, 41, and subgenus F are responsible for the majority of adenovirus-mediated cases of gastroenteritis. Pathogenicity of the virus varies with the species and serotype, and organ specificity and disease patterns appear to be serotype-dependent.
Human adenoviruses are transmitted by the fecal–oral route and through inhalation of water droplets. They are listed as pathogens of childhood gastroenteritis as most affected are being children of under 5 years of age. AdV resistance to purification and disinfection processes (i.e., UV inactivation) and the virus's ability to survive in the environment have increased the importance of monitoring AdVs in water ( Jiang, 2006 ). Theses adenoviruses are being documented everywhere in the world, without any seasonal variability and could be found in drinking water if not properly treated, in raw sewage, polluted waters such as rivers and dams, swimming pools, and even in shellfish.
2.2.2. Astroviruses
AstVs are nonenveloped, icosahedral viruses belonging to family Astroviridae . They are 28–41 nm in size, containing positive-sense, single-stranded RNA, genome of approximately 7 kb in size. They have been classified into two genera: Mamastrovirus and Avastrovirus . Genera Mamastrovirus and Avastrovirus cause infection to mammalians and avian, respectively. Three divergent groups of human astroviruses (HAstVs) have been identified and according to research, the classic AstV group contains eight serotypes accounting for less than 10% of all acute nonbacterial gastroenteritis in children worldwide without any geographical boundaries. Children get infected in the first few years of life regardless of their level of hygiene, quality of water, food or sanitation, or type of behavior. Although children are vulnerable, there are reports of disease in normal healthy adults ( Pager and Steele, 2002 ) and also immunocompromised individuals ( González et al., 1998 ). This infection induces mild, watery diarrhea that lasts 2–3 days, associated with vomiting, fever, anorexia, and abdominal pain. In comparison to RV or calicivirus infection, infections due to AstVs have a longer incubation period.
These viruses can be transmitted by the contaminated water via the fecal–oral route, and higher incidence has been recorded in cold months. In temperate regions, most AstV infections are during winter, whereas in tropics, infections occur during rainy months. These viruses are being detected both in surface and groundwaters which are being used as drinking water sources, freshwater, and marine waters as well as in wastewater effluents. Moreover, waterborne transmission of HAstVs has been suggested as a risk of digestive morbidity for the general population ( Gofti-Laroche et al., 2003 ). Chlorine and other disinfectants are effective for the inactivation of these viruses in water.
2.2.3. Caliciviruses
Caliciviruses are nonenveloped, 27–40 nm single-stranded RNA viruses in the family Caliciviridae. They are an important group of human viruses capable of causing gastrointestinal disease in humans that may be found in waters intended by humans for drinking, recreation, and shellfish growing. The International Committee on Taxonomy of Viruses changed the calicivirus nomenclature and classified into four genera: Vesivirus , Lagovirus , Norovirus , and Sapovirus . Sapoviruses (SaVs) and noroviruses (NoVs) are included in the latest US Drinking Water Contaminant Candidate List (CCL) ( Rusinol and Girones, 2017 ). They get spread by the fecal–oral route and are found in contaminated surface and groundwaters. The presence of caliciviruses in groundwaters is an important consideration, as a number of outbreaks have been linked to these drinking water sources (often from shallow wells and springs) ( Schaub and Oshiro, 2000 ).
2.2.4. Noroviruses
Noroviruses (NoVs) (formerly Norwalk virus) were first identified following an outbreak of enteric illness among children and adults in the town of Norwalk, Ohio ( Adler and Zickl, 1969 ). They are a group of nonenveloped, single-stranded RNA viruses with an icosahedral symmetry classified into the genus Norovirus of the family Caliciviridae with a size of 27–32 nm. Most norovirus genomes contain three open reading frames (ORFs). They have rough, nondistinct borders and lack the calyx appearance. Noroviruses are divided into five genogroups (GI to GV), three of which (GI, GII, and GIV) cause human disease. This virus is extremely infectious and humans are the only known reservoir for human norovirus. NoVs cause acute onset of projectile vomiting and diarrhea, sometimes with low-grade fever, headache, and malaise. Symptoms are usually self-limited, lasting for 24–72 h. The incubation period is usually 24–48 h, but onset of symptoms as soon as 10 h after exposure has been reported. Disease outbreaks have been associated with consumption of these viruses in drinking water and also in contaminated shellfish ( Maunula et al., 2005 , Boxman et al., 2006 ).
2.2.5. Sapoviruses
Sapovirus (SaV) is one of the etiological agents of human gastroenteritis, is named after the Japanese city Sapporo, where it was first discovered ( Chiba et al., 1979 ). SaV is an RNA virus with a nonsegmented, positive-sense, single-stranded RNA molecule of approximately 7.3–7.5 kb, belonging to the family Caliciviridae. Genome organization of SaVs differs to NoV and contains only two ORFs instead of three. SaVs have a nonenveloped viral capsid with icosahedral symmetry and display a characteristic surface that has cup-shaped depressions on the surface, formed by the 32 cups or “calices,” which is a typical calicivirus morphology. SaVs show a high level of diversity in their genomes and are currently divided into at least five genetically distinct genogroups. Infections in humans are caused by viruses of genogroup GI, GII, GIV, and GV, and at present human SaV genogroups are classified into 16 genotypes. The disease outbreaks are reported in all age groups including the elderly people ( Lee et al., 2012 ). SaVs are transmitted from person to person via fecal–oral routes and through contaminated foods and water.
2.2.6. Enteroviruses
The waterborne polioviruses, coxsackieviruses, echoviruses, hepatoviruses, and certain unclassified enteroviruses together as a group named as enteroviruses are belonging to the family Picornaviridae. They are small in size, 22–30 nm in diameter, and nonenveloped, and the virions are relatively simple, consisting of a protein capsid surrounding a single-stranded, positive-sense RNA genome and it is assumed that only reservoir for enteroviruses are humans. They are present mainly in sewage contaminated with human feces but can be found in groundwaters, coastal river and marine waters, sewage treatment plants and from solid waste landfills, and insufficiently treated drinking water.
These have been identified as sensitive to formaldehyde, hydroxylamine, UV, ionizing irradiations, and also to ozone but cannot be inactivated with changing pH or with usual chlorination. It is assumed that infections from enteroviruses are associated with poverty and poor hygienic conditions.
2.2.7. Hepatovirus A
Hepatovirus A (HAV) is a nonenveloped virus with an icosahedral capsid of about 27–32 nm, single-stranded having an RNA genome of approximately 7.5 kb and belongs to the family Picornaviridae. HAV is the causative agent of type A viral hepatitis and only one serotype has been reported ( Cristina and Costa-Mattioli, 2007 ). Virus transmission occurs through the fecal–oral route by direct contact with an infected person or exposure to contaminated water or consumption of contaminated food.
The incidence of HAV shows distinct patterns of geographic distribution and being related to standards of hygiene and sanitation, demographic factors and socioeconomic conditions of the population. Most of the infections occur in Africa and Asia, followed by Central and South America, Eastern Europe are considered as areas of intermediate endemicity. Children are more vulnerable to disease in highly endemic areas while adolescents and adults are susceptible to infection in intermediate endemic areas ( WHO, 2012 ).
2.2.8. Hepatovirus E
Hepatovirus E (HEV) is a nonenveloped, positive-sense, single-stranded RNA genome of 7.2 kb in length and belongs to the family Hepeviridae. Family Hepeviridae contains two genera: Orthohepevirus and Piscihepevirus . Four main genotypes of HEV (HEV-1, HEV-2, HEV-3, and HEV-4) belonging to the Orthohepevirus A species are able to infect humans. HEV is primarily transmitted by fecal–oral routes through contaminated foods and water in endemic areas. Also, zoonotic and person-to-person transmission is possible. HEV causes acute hepatitis E in human and an infection is considered to be endemic in many developing countries in Africa and Asia. HEV genotypes 1 and 2 cause epidemic and endemic diseases in developing countries, mainly through contaminated drinking water, while genotypes 3 and 4 cause autochthonous infections mainly in developed countries through a unique zoonotic foodborne transmission ( Khuroo et al., 2016 ).
2.2.9. Rotaviruses
RVs are nonenveloped, double-stranded RNA viruses, belonging to the family Reoviridae. RV is composed of the outer capsid, inner capsid, and core, and genome is composed of 11 segments of double-stranded RNA, which code for six structural and five nonstructural proteins. RV is mainly classified into seven groups (A–G) based on the antigenicity of the inner capsid protein VP6 and genomic characteristics. Among them, rotavirus group A (RVA) strains with distinct G-genotype and P-genotype are the major etiological agents in humans worldwide. Infection with RVA is the most common cause of diarrheal disease among infants and young children and one of the common causes of death in children under 5 years of age ( Walker et al., 2013 ). Virus transmission occurs through the fecal–oral route by direct contact with an infected person and possibly by the respiratory route. RV causes an estimated 2 million hospitalizations and 450,000 deaths among children annually, and the majority of deaths are reported from developing countries in Asia and Africa ( Wang et al., 2014 , Liu et al., 2015 ). The WHO has recommended that the use of RV vaccines in routine immunization programs worldwide to reduce the burden of disease ( WHO, 2009 ).
2.3. Waterborne protozoa
Protozoan parasites were the most frequently identified etiologic agents in WBDOs in 1990s. Further from 1978 through 1991, Giardia lamblia was the most commonly identified pathogen, while in 1992, the numbers of outbreaks reported for giardiasis and cryptosporidiosis were matching. Naegleria fowleri, Acanthamoeba spp., and Entamoeba histolytica are also considered as etiologic agents in WBDOs. Since the potential threat of infection via the waterborne route is being recognized for many of these protozoans, it is crucial that the water industry pays its attention to finding ways to detect these emerging and well-recognized protozoan pathogens in water ( Marshall et al., 1997 ).
2.3.1. The genus Cryptosporidium
Cryptosporidia are zoonotic protozoan parasites with worldwide distribution, consisting of 27 species and more than 60 genotypes. Among Cryptosporidium species identified, Cryptosporidium hominis and Cryptosporidium parvum are the major disease-causing organisms in human. They cause cryptosporidiosis which is a gastrointestinal illness that can last for several days to several weeks. This infection is commonly found in children, immunocompromised individuals, and workers who are frequently exposed to wastewater. The major routes of transmission are not only water and food but also person-to-person contact and respiratory transmission is possible.
2.3.2. The genus Giardia
Giardia are flagellated protozoan parasites belonging to the phylum Metamonada that cause giardiasis, a diarrheal disease in humans and other mammals throughout the world. Since 1920, six Giardia species have been described; Giardia duodenalis (syn. Giardia intestinalis and G . lamblia ) is the major disease-causing organisms in human. Risk from Giardia can be through occupational, accidental, or recreational exposure to surface waters. Brodsky et al. (1974) reported that water contaminated with G. lamblia cysts causes travel-related giardiasis in tourists in certain areas of the world. Giardia species have two major stages in their life cycle, i.e., rapidly multiplying trophozoites and cysts. Cysts are excreted with feces and survive in a variety of environmental conditions. They can be transmitted through contaminated water, food, or direct fecal–oral route.
2.3.3. Entamoeba histolytica
E . histolytica belongs to the family Entamoebidae, an invasive, pathogenic protozoan causing amebiasis while other two species Entamoeba dispar and Entamoeba moshkovskii are nonpathogenic. Life cycle of this E . histolytica includes trophozoite, precyst, cyst, metacyst, and metacystic trophozoite stages. Mature cysts have four nuclei and average 20 μm in diameter, while the motile form trophozoite has a size range of 10–60 μm. The cyst form is the infective form for humans, which can survive in water and food. Infections due to E. histolytica have been recorded globally and it is suggested that from the infected persons around 10% show clinical symptoms. According to literature except for the two parasites, plasmodia and schistosomes, most deaths have been assigned to E. histolytica than any other parasite. In developed countries, risk groups include travelers, immigrants, migrant workers, and immunocompromised individuals. Transmission of this protozoan by water is common in developing countries, where much of the water supply for drinking is untreated and fecally contaminated ( Marshall et al., 1997 ).
2.4. Waterborne helminths
The helminths, generally known as parasitic worms, are invertebrates with elongated, flat, or round bodies which belong to Kingdom Animalia. The major parasitic helminths include in the phylum Nematoda (roundworms) and the phylum Platyhelminthes (trematodes). Helminth parasites infect a large number of people and animals worldwide, mainly in developing countries due to lack of water, sanitation, and hygiene facilities. Dracunculus medinensis (Guinea worm) and Fasciola spp. ( Fasciola hepatica and Fasciola gigantica ) (liver flukes) are the major helminths which can be transmitted through drinking water.
2.4.1. The genus Dracunculus
The genus Dracunculus belongs to the phylum Nematoda and family Dracunculidae, which is parasite of mammals and reptiles. There are 14 valid species in this genus but D. medinensis has been well-studied because of human infections. Dracunculiasis or Guinea-worm disease (GWD) is an avoidable waterborne disease caused by the parasite D. medinensis which affect the populations in rural parts of South Asia and Africa. Reported cases worldwide annually have declined from an estimated 3.5 million cases in 1986 to only 28 cases in 2018 ( WHO, 2019 ). GWD is now restricted to some communities in remote parts of Africa. Humans get exposed to the disease through consumption of drinking water containing Cyclops spp. carrying infectious D. medinensis larvae. After ingestion, larvae are released, penetrate the intestinal and peritoneal walls, and inhabit the subcutaneous tissues.
2.4.2. The genus Fasciola
The genus Fasciola belongs to the phylum Platyhelminthes and family Fasciolidae, which causes fasciolosis in human and ruminants. The main pathogenic species are F . hepatica (temperate fluke) and F . gigantica (tropical fluke). It is estimated that more than 17 million people are infected worldwide and about 180 million people living in endemic areas are at risk to infection ( Cwiklinski et al., 2016 ). Human infection generally occurs through consumption of aquatic vegetables such as watercress, drinking water contaminated with encysted cercariae, or washing utensils with contaminated water.
The above-discussed and the most important waterborne pathogens belonging to the four categories bacteria, viruses, protozoa, and helminths, their diseases, and mode of transmission are summarized in Table 2.1 .
The waterborne pathogens.
3. Potential waterborne pathogens
In 1997 , WHO defined emerging pathogens as those that have appeared in a human population for the first time or have occurred previously but are increasing in incidence or expanding into geographical areas where they have not previously been reported. Reemerging pathogens are those whose occurrence is increasing as a result of long-term changes in their underlying epidemiology ( WHO, 2003 ). By these criteria, 175 species of infectious agent from 96 different genera were classified as emerging pathogens in 1970s and from this group, 75% were zoonotic species. However, currently several of this microorganism from environmental sources, including water, have been confirmed as pathogens, including Cryptosporidium , Legionella , E. coli O157, RV, hepatitis E virus, and norovirus. H . pylori is an example of a recently emerged pathogen that may be transmitted through water ( WHO, 2003 ).
3.1. Potential waterborne bacteria
3.1.1. helicobacter pylori.
Bacteria of genus Helicobacter are Gram-negative, curved, or spiral-shaped belonging to the family Helicobacteraceae and class Epsilonproteobacteria . H . pylori is a helix-shaped bacterium, 3 μm long with a diameter about 0.5 μm. Genus Helicobacter contains more than 40 described species and 4 Candidatus species, a designation of provisional status by International Committee on Systematic Bacteriology for incompletely described prokaryotes, and is divided according to their major colonization sites as gastric or lower intestinal tract–associated bacterial species. It is a genetically diverse gastric pathogen, carrying a range of antibiotic resistance patterns, and varies in geographic occurrence.
These are considered as major etiologic agent for gastritis and are also connected to pathogenesis of peptic and duodenal ulcer disease and gastric carcinoma. But most individuals remain asymptomatic. Approximately 70%–90% of persons in developing countries and 25%–50% of those in developed countries are colonized by H. pylori and it is transmitted mainly by fecal–oral or oral–oral routes, with water and food as the sources ( Doyle, 2012 ). Epidemiological studies have associated the H. pylori infection with lack of access to potable drinking water and proper sanitation H. pylori in drinking water biofilms, change their morphology, and persist for more than 1 month, with densities exceeding 10 6 cells/cm 2 ( Giao et al., 2008 ).
3.1.2. Aeromonas hydrophila
Aeromonas are straight, coccobacillary to bacillary, belonging to family Aeromonadaceae of order Aeromonadales who are non–spore-forming, facultative anaerobic, Gram-negative bacteria with cells having a size of 0.3–1.0 × 1.0–3.5 μm. Although Aeromonas hydrophila is usually the dominant species, other aeromonads, such as Aeromonas caviae and Aeromonas sobria , have also been isolated from human feces and water sources.
A. hydrophila has been recognized as an opportunistic pathogen being identified as a potential agent of gastroenteritis, septicemia, meningitis, and in wound infections. It plays a significant role in intestinal disorders in children under 5 years old, the elderly, and immunosuppressed people. Ubiquitous in nature, it is frequently isolated from food, drinking water, and aquatic environments. In surface waters, mainly rivers and lakes, concentrations of Aeromonas spp. are high but groundwaters generally contain lesser numbers. Drinking water immediately leaving the treatment plant could contain between 0 and 10 2 CFU/mL and these waters can display higher Aeromonas concentrations, due to the growth in biofilms ( Chauret et al., 2001 ). A. hydrophila is resistant to standard chlorine treatments and it is assumed that they survive by being within the biofilms. The common routes of infection are the ingestion of contaminated water or food or through skin. No person-to-person transmission has been reported.
3.1.3. The genus Leptospira
The genus Leptospira belongs to family Leptospiraceae of the phylum Spirochaete and currently contains 20 species including 9 pathogenic, 6 saprophytic, and 5 being intermediate. They are thin, tightly coiled, motile spirochetes, generally 6–20 mm in length, but during culturing they may produce much longer cells. The surface structure of the Leptospira shows both Gram-negative and Gram-positive characteristics. The disease leptospirosis is one of the most widespread zoonotic diseases, infecting both human and animals caused by the Leptospira . The major route of exposure to the pathogen is indirect contact with contaminated water or moist soil. In developing countries from tropics, leptospirosis is an occupational infection, most affected being the people who are engaged in farming, sharecropping, and in animal husbandry ( Levett, 2001 ). Furthermore, there is a significant risk of exposure during recreational activities. Leptospirosis is essentially waterborne infection, as several outbreaks of disease have been recorded during rainy season. Both pathogenic and saprophytic strains of leptospirosis have been isolated from water sources including rivers and lakes as they are able to survive in moist soil and freshwater for long periods of time ( Pal and Hadush, 2017 ).
3.1.4. The genus Tsukamurella
Bacteria belonging to genus Tsukamurella of order Actinomycetales are Gram-positive, nonmotile, obligate aerobic, irregular, and rod-shaped. The genus includes 11 species and out of which 9 Tsukamurella species have been isolated from human infections. Most of Tsukamurella species exist as environmental saprophytes present in soil, arthropods, water, sludge foam, and sponges. Some species have been detected in drinking water supplies, but there is no evidence of correlation between the presence of organisms and the disease. They are opportunistic pathogens and can transmit through clinical instruments such as catheters or lesions. Tsukamurella cause various infections in humans, including pulmonary and cutaneous infections and meningitis and most vulnerable are immunocompromised individuals.
3.1.5. The genus Bacillus
Bacteria belonging to genus Bacillus of phylum Firmicutes are rod-shaped, Gram-positive, strictly aerobic, or facultatively anaerobic and are capable of endospore formation. Bacillus species are commonly found in soil and water. They have been detected in drinking water supplies even after disinfection processes, but waterborne transmission is not yet confirmed. Only few Bacillus species are pathogenic to human. With Bacillus cereus causing bacteremia in immunocompromised patients, B. anthracis causes anthrax in humans and animals. In a study conducted by Taylor et al. (2005) , strains of Bacillus megaterium, Bacillus firmus , Bacillus simplex , and B. cereus were found to produce heat-stable toxins, with varying levels of toxicity.
3.1.6. Cyanobacteria and cyanotoxins
Cyanobacteria are a phylum with an estimated 150 genera of cyanobacteria containing approximately 2000 species, of which around 46 have been reported as being toxigenic. They are oxygenic, photosynthetic, Gram-negative bacteria that inhabit a large variety of terrestrial and aquatic habitats, showing a wide diversity in morphology and their average cell size ranges from 0.5 to 60 μm. In 1998, cyanobacteria were included as a microbial contaminant to CCL because of their potential for transmission through drinking water. Furthermore, microcystin-LR, cylindrospermopsin, and anatoxin-a produced by several species of cyanobacteria are also included in the CCL. These cyanotoxins have been reported from water reservoirs around the world which had caused acute and chronic illnesses in animals and humans ( Liyanage et al., 2016 ). Exposure to cyanotoxins can be through contaminated drinking water, ingestion and dermal skin contact during recreational activities ( Fig. 2.2 ), inhalation of aerosols, medical treatments (dialysis), or through algal food supplements.
Figure 2.2.
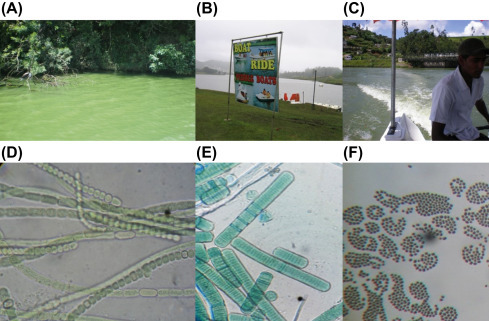
A lake in a developing country where people are engaged in recreational activities. (A) Lake Gregory, Sri Lanka; (B) recreational activity area; (C) people engaged in boat riding; potential toxic cyanobacteria; (D) Anabaena sp.; (E) Oscillatoria sp.; (F) Microcystis sp. ( Magana-Arachchi et al., 2011 ).
3.2. Potential waterborne viruses
In 2017, GWPP reported 10 emerging viruses with potential for waterborne transmission including genera Alphatorquevirus , Cyclovirus , Erythroparvovirus , Bocaparvovirus , Protoparvovirus , Alphapapillomavirus , Betapapillomavirus , Picobirnavirus , Betapolyomavirus , and Alphapolyomavirus ( Rusinol and Girones, 2017 ) ( Table 2.2 ).
The potential waterborne pathogens.
Adapted from Table 2.2 in summary of excreted and waterborne viruses ( Rusinol and Girones, 2017 ).
3.3. Potential waterborne protozoa
3.3.1. microsporidia.
Microsporidia belonging to the phylum Microspora include over 140 genera and 1200 species that are parasitic in all major animal groups. They are obligate intracellular, spore-forming protists. The spore is the only stage that can survive outside the host cell in their life cycle and it contains a characteristic coiled polar filament for injecting the sporoplasm into a host cell to initiate infection. After infection, a complex process of multiplication takes place within an infected cell and new spores are produced and released to feces, urine, respiratory secretions, or other body fluids, depending on the type of species and the site of infection. Among 14 human pathogenic Microsporidia species, two species, Enterocytozoon bieneusi and Encephalitozoon intestinalis , are the most prevalent species which associated with gastrointestinal disease in humans. Person-to-person contact and ingestion of spores in water and food contaminated with human feces or urine are considered as important routes of exposure. A study by Dowd et al. (1998) showed that 7 out of 14 water concentrates tested were contaminated with E. intestinalis , E. bieneusi , and Vittaforma corneae which represent human pathogenic microsporidia species. Their study is the first species level confirmation of human pathogenic microsporidia in water, indicating that these human pathogenic microsporidia possibly be waterborne pathogens. Microsporidiosis is an emerging disease in immunosuppressed persons with AIDS, but microsporidia have the ability to cause disease even in immunologically normal hosts.
3.3.2. Cystoisospora belli
Cystoisospora (formerly Isospora ) are coccidian parasites, belonging to the phylum Apicomplexa, found mainly in tropical and subtropical areas. Many Cystoisospora species can infect animals, but human is the only known host for C . belli ( Lindsay et al., 1997 ) . C. belli infects the epithelial cells of the small intestine of human, and immunocompromised individuals are more susceptible to the infection. The immature form of the parasite is known as oocytes; they are passed out with feces and then mature outside the body in 2–3 days, depending on environmental conditions. It can be transmitted through contaminated water and food. However, direct person-to-person transmission is unlikely. The improved practice of personal hygiene and sanitation may help in preventing transmission of disease.
3.3.3. Cyclospora cayetanensis
Cyclospora cayetanensis belongs to the family Eimeriidae, 7.5–10 μm in diameter, cyst-forming, and unsporulated when passed in feces. It is an apicomplexa coccidia closely related to Eimeria species, recognized as an emerging protist that causes diarrheal illness and significantly contributes to the burden of gastroenteritis worldwide.
3.4. Potential waterborne helminths
3.4.1. the genus schistosoma.
Schistosomes are trematode parasites which cause schistosomiasis (or bilharzia) in human. The main human pathogenic species are Schistosoma mansoni , Schistosoma japonicum , and Schistosoma haematobium . Schistosomiasis is a waterborne disease mostly seen in the tropics and subtropics. The humans get exposed when their skin comes into contact with infested freshwater, into which the cercariae of the parasite are released by freshwater snails. It is understood that for each of the human schistosomes, the presence of a specific genus of snail is necessary for transmission to occur. This disease has been considered as a disease due to poverty, and controlling of this disease has been a problem because of the lack of clean water available to people living in the developing countries.
The most important potential waterborne pathogens as described by WHO belonging to the four categories bacteria, viruses, protozoa, and helminths, their diseases, and mode of transmission are summarized in Table 2.2 .
This chapter provides a general description on current waterborne pathogens as well as emerging and potential pathogens which could be categorized into bacteria, viruses, protozoans, and helminths. Most of these microorganisms are ubiquitous in waters regardless of ground, surface, fresh, or marine. People utilize these waters for drinking, cooking, and other domestic actions, bathing, medically, and also for recreations. The water sources become polluted due to the mixing of fecal matter from human and animal and also waste generated by other direct and indirect anthropogenic activities. As a result, waters become reservoirs for the pathogens making it unsafe for human consumption causing many waterborne diseases. In addition, with the increase in global population, changes in climatic patterns, and the presence of antibiotic resistant bacteria in waste waters, it is predicted that there will be a rise in waterborne diseases especially diarrhea and cholera. Therefore, still the global populations infants, young, or old are at a risk from waterborne diseases and outbreaks whether the countries are developed or developing or in tropics or temperate in geographical distribution. Hence to minimize the adverse effects from these waterborne pathogens and to improve the water quality, regular monitoring of water sources is essential with advanced but cost-effective detection techniques, precise disinfectant procedures with proper management.
Acknowledgment
We are expressing our sincere gratitude to Ms. Chanusha Weralupitiya for technical support in preparation of Fig. 2.1 .
- Adler L., Zickl R. Winter vomiting disease. J. Infect. Dis. 1969;119(6):668–673. doi: 10.1093/infdis/119.6.668. [ DOI ] [ PubMed ] [ Google Scholar ]
- Aissa R., Al-Gallas N., Troudi H., Belhadj N., Belhadj A. Trends in Salmonella enterica serotypes isolated from human, food, animal, and environment in Tunisia, 1994–2004. J. Infect. 2007;55(4):324–339. doi: 10.1016/j.jinf.2007.06.007. [ DOI ] [ PubMed ] [ Google Scholar ]
- Arvanitidou M., Kanellou K., Vagiona D. Diversity of Salmonella spp. and fungi in northern Greek rivers and their correlation to fecal pollution indicators. Environ. Res. 2005;99(2):278–284. doi: 10.1016/j.envres.2005.01.002. [ DOI ] [ PubMed ] [ Google Scholar ]
- Bivins A., Sumner T., Kumpel E., Howard G., Cumming O., Ross I., Nelson K., Brown J. Estimating infection risks and the global burden of diarrheal disease attributable to intermittent water supply using QMRA. Environ. Sci. Technol. 2017;51(13):7542–7551. doi: 10.1021/acs.est.7b01014. [ DOI ] [ PubMed ] [ Google Scholar ]
- Boxman L., Tilburg J., TeLoeke A., Vennema H., Jonker K., De Boer E., Koopmans M. Detection of noroviruses in shellfish in The Netherlands. Int. J. Food Microbiol. 2006;108(3):391–396. doi: 10.1016/j.ijfoodmicro.2006.01.002. [ DOI ] [ PubMed ] [ Google Scholar ]
- Bridle H. Overview of waterborne pathogens. In: Bridle H., editor. Waterborne Pathogens: Detection Methods and Applications. Academic Press; 2013. [ Google Scholar ]
- Brodsky E., Spencer C., Schultz G. Giardiasis in American travelers to the Soviet Union. J. Infect. Dis. 1974;130(3):319–323. doi: 10.1093/infdis/130.3.319. [ DOI ] [ PubMed ] [ Google Scholar ]
- Bruneau A., Rodrigue H., Ismael J., Dion R., Allard R. Outbreak of E. coli O157:H7 associated with bathing at a public beach in the Montreal-Centre region. Can. Commun. Dis. Rep. 2004;30(15):133–136. [ PubMed ] [ Google Scholar ]
- Cabral J. Water microbiology. Bacterial pathogens and water. Int. J. Environ. Res. Public Health. 2010;7(10):3657–3703. doi: 10.3390/ijerph7103657. [ DOI ] [ PMC free article ] [ PubMed ] [ Google Scholar ]
- Chauret C., Volk C., Creason R., Jarosh J., Robinson J., Warnes C. Detection of Aeromonas hydrophila in a drinking-water distribution system: a field and pilot study. Can. J. Microbiol. 2001;47(8):782–786. [ PubMed ] [ Google Scholar ]
- Chiba S., Sakuma Y., Kogasaka R., Akihara M., Horino K., Nakao T., Fukui S. An outbreak of gastroenteritis associated with calicivirus in an infant home. J. Med. Virol. 1979;4(4):249–254. doi: 10.1002/jmv.1890040402. [ DOI ] [ PubMed ] [ Google Scholar ]
- Colquhoun J., Larsson P., Duodu S., Forsman M. The family Francisellaceae. In: Rosenberg E., DeLong E.F., Lory S., Stackebrandt E., Thompson F., editors. The Prokaryotes. Springer; Berlin, Heidelberg: 2014. [ Google Scholar ]
- Corea E., Thevanesam V., Perera S., Jayasinghe I., Ekanayake A., Masakorala J., Inglis T. Melioidosis in Sri Lanka: an emerging infection. Sri Lankan J. Infect. Dis. 2012;1(2):2–8. [ Google Scholar ]
- Cristina J., Costa-Mattioli M. Genetic variability and molecular evolution of hepatitis A virus. Virus Res. 2007;127(2):151–157. doi: 10.1016/j.virusres.2007.01.005. [ DOI ] [ PubMed ] [ Google Scholar ]
- Currie B., Dance D., Cheng A. The global distribution of Burkholderia pseudomallei and melioidosis: an update. Trans. R. Soc. Trop. Med. Hyg. 2008;102(S1):S1–S4. doi: 10.1016/S0035-9203(08)70002-6. [ DOI ] [ PubMed ] [ Google Scholar ]
- Cwiklinski K., O'neill S.M., Donnelly S., Dalton J.P. A prospective view of animal and human fasciolosis. Parasite Immunol. 2016;38(9):558–568. doi: 10.1111/pim.12343. [ DOI ] [ PMC free article ] [ PubMed ] [ Google Scholar ]
- Dowd E., Gerba P., Pepper L. Confirmation of the human-pathogenic microsporidia Enterocytozoon bieneusi, Encephalitozoon intestinalis, and Vittaforma corneae in water. Appl. Environ. Microbiol. 1998;64(9):3332–3335. doi: 10.1128/aem.64.9.3332-3335.1998. [ DOI ] [ PMC free article ] [ PubMed ] [ Google Scholar ]
- Doyle E. University of Wisconsin; Madison: 2012. Helicobacter spp.- Food- or Waterborne Pathogens? FRI Food Safety Review. [ Google Scholar ]
- Giao M., Azevedo N., Wilks S., Vieira M., Keevil C. Persistence of Helicobacter pylori in heterotrophic drinking-water biofilms. Appl. Environ. Microbiol. 2008;74(19):5898–5904. doi: 10.1128/AEM.00827-08. [ DOI ] [ PMC free article ] [ PubMed ] [ Google Scholar ]
- Gibson K.E. Viral pathogens in water: occurrence, public health impact, and available control strategies. Curr. Opin. Virol. 2014;4:50–57. doi: 10.1016/j.coviro.2013.12.005. [ DOI ] [ PMC free article ] [ PubMed ] [ Google Scholar ]
- Gofti-Laroche L., Gratacap-Cavallier B., Demanse D., Genoulaz O., Seigneurin J., Zmirou D. Are waterborne astrovirus implicated in acute digestive morbidity (E.MI.R.A. study)? J. Clin. Virol. 2003;27(1):74–82. doi: 10.1016/s1386-6532(02)00130-0. [ DOI ] [ PubMed ] [ Google Scholar ]
- González G., Pujol H., Liprandi F., Deibis L., Ludert E. Prevalence of enteric viruses in human immunodeficiency virus seropositive patients in Venezuela. J. Med. Virol. 1998;55(4):288–292. doi: 10.1002/(sici)1096-9071(199808)55:4<288::aid-jmv6>3.0.co;2-x. [ DOI ] [ PubMed ] [ Google Scholar ]
- Jiang C. Human adenoviruses in water: occurrence and health implications: a critical review. Environ. Sci. Technol. 2006;40(23):7132–7140. doi: 10.1021/es060892o. [ DOI ] [ PubMed ] [ Google Scholar ]
- Jin D., Kong X., Cui B., Jin S., Xie Y., Wang X., Deng Y. Bacterial communities and potential waterborne pathogens within the typical urban surface waters. Sci. Rep. 2018;8(1):13368. doi: 10.1038/s41598-018-31706-w. [ DOI ] [ PMC free article ] [ PubMed ] [ Google Scholar ]
- Khuroo M., Khuroo M., Khuroo N. Transmission of hepatitis E virus in developing countries. Viruses. 2016;8(9):253. doi: 10.3390/v8090253. [ DOI ] [ PMC free article ] [ PubMed ] [ Google Scholar ]
- Lee E., Cebelinski A., Fuller C., Keene E., Smith K., Vinjé J., Besser M. Sapovirus outbreaks in long-term care facilities, Oregon and Minnesota, USA, 2002-2009. Emerg. Infect. Dis. 2012;18(5):873–876. doi: 10.3201/eid1805.111843. [ DOI ] [ PMC free article ] [ PubMed ] [ Google Scholar ]
- Levett N. Leptospirosis. Clin. Microbiol. Rev. 2001;14(2):296–326. doi: 10.1128/CMR.14.2.296-326.2001. [ DOI ] [ PMC free article ] [ PubMed ] [ Google Scholar ]
- Limmathurotsakul D., Kanoksil M., Wuthiekanun V., Kitphati R., Day P., Peacock J. Activities of daily living associated with acquisition of melioidosis in northeast Thailand: a matched case-control study. PLoS Neglected Trop. Dis. 2013;7(2):2072. doi: 10.1371/journal.pntd.0002072. [ DOI ] [ PMC free article ] [ PubMed ] [ Google Scholar ]
- Lindsay S., Dubey P., Blagburn L. Biology of Isospora spp. from humans, nonhuman primates, and domestic animals. Clin. Microbiol. Rev. 1997;10(1):19–34. doi: 10.1128/cmr.10.1.19. [ DOI ] [ PMC free article ] [ PubMed ] [ Google Scholar ]
- Liu L., Oza S., Hogan D., Perin J., Rudan I., Lawn J.E., Cousens S., Mathers C., Black R.E. Global, regional, and national causes of child mortality in 2000-13, with projections to inform post-2015 priorities: an updated systematic analysis. Lancet. 2015;385(9966):430–440. doi: 10.1016/S0140-6736(14)61698-6. [ DOI ] [ PubMed ] [ Google Scholar ]
- Liyanage H.M., Magana Arachchi D.N., Abeysekara T., Guneratne L. Toxicology of freshwater cyanobacteria. J. Environ. Sci. Health Part C. 2016;34(3):137–168. doi: 10.1080/10590501.2016.1193923. [ DOI ] [ PubMed ] [ Google Scholar ]
- Magana-Arachchi D.N., Wanigatunge R.P., Liyanage H.M. Molecular characterization of cyanobacterial diversity in Lake Gregory, Sri Lanka. Chin. J. Oceanol. Limnol. 2011;29(4):898–904. [ Google Scholar ]
- Marshall M.M., Naumovitz D., Ortega Y., Sterling C.R. Waterborne protozoan pathogens. Clin. Microbiol. Rev. 1997;10(1):67–85. doi: 10.1128/cmr.10.1.67. [ DOI ] [ PMC free article ] [ PubMed ] [ Google Scholar ]
- Maunula L., Miettinen T., Von Bonsdorff H. Norovirus outbreaks from drinking water. Emerg. Infect. Dis. 2005;11(11):1716–1721. doi: 10.3201/eid1111.050487. [ DOI ] [ PMC free article ] [ PubMed ] [ Google Scholar ]
- Murray J., Vos T., Lozano R., Naghavi M., Flaxman D., Michaud C., Ezzati M., Shibuya K., Salomon A., Abdalla S., Aboyans V. Disability-adjusted life years (DALYs) for 291 diseases and injuries in 21 regions, 1990–2010: a systematic analysis for the global burden of disease study 2010. Lancet. 2012;380(9859):2197–2223. doi: 10.1016/S0140-6736(12)61689-4. [ DOI ] [ PubMed ] [ Google Scholar ]
- Pager T., Steele D. Astrovirus-associated diarrhea in South African adults. Clin. Infect. Dis. 2002;35(11):1452–1453. doi: 10.1086/344456. [ DOI ] [ PubMed ] [ Google Scholar ]
- Pal M., Hadush A. Leptospirosis: an infectious emerging waterborne zoonosis of global significance. Air Water Borne Dis. 2017;6:1–4. [ Google Scholar ]
- Patel C., Shanker R., Gupta V., Upadhyay R. Q-PCR based culture-independent enumeration and detection of Enterobacter: an emerging environmental human pathogen in riverine systems and potable water. Front. Microbiol. 2016;7:172. doi: 10.3389/fmicb.2016.00172. [ DOI ] [ PMC free article ] [ PubMed ] [ Google Scholar ]
- Rusinol M., Girones R. Summary of excreted and waterborne viruses. In: Rose J.B., Jiménez-Cisneros B., editors. Global Water Pathogen Project (GWPP) Michigan State University; E. Lansing, MI: 2017. UNESCO. [ Google Scholar ]
- Schaub S., Oshiro R. Public health concerns about Caliciviruses as waterborne contaminants. J. Infect. Dis. 2000;181(s2):S374–S380. doi: 10.1086/315580. [ DOI ] [ PubMed ] [ Google Scholar ]
- Taylor J.M., Sutherland A.D., Aidoo K.E., Logan N.A. Heat-stable toxin production by strains of Bacillus cereus, Bacillus firmus, Bacillus megaterium, Bacillus simplex and Bacillus licheniformis. FEMS Microbiol. Lett. 2005;242(2):313–317. doi: 10.1016/j.femsle.2004.11.022. [ DOI ] [ PubMed ] [ Google Scholar ]
- United Nations General Assembly (UNGA) 2010. The Human Right to Water and Sanitation: Resolution/Adopted by the General Assembly. [ Google Scholar ]
- UNSD . United Nations General Assembly Resolution (UNSD); 2015. Transforming Our World: The 2030 Agenda for Sustainable Development. A/RES/70/1. [ Google Scholar ]
- Walker F., Rudan I., Liu L., Nair H., Theodoratou E., Bhutta A., O'Brien L., Campbell H., Black E. Global burden of childhood pneumonia and diarrhoea. Lancet. 2013;381(9875):1405–1416. doi: 10.1016/S0140-6736(13)60222-6. [ DOI ] [ PMC free article ] [ PubMed ] [ Google Scholar ]
- Wang H., Liddell A., Coates M., Mooney D., Levitz E., Schumacher E., Murray C.J. Global, regional, and national levels of neonatal, infant, and under-5 mortality during 1990–2013: a systematic analysis for the global burden of disease study 2013. Lancet (Lond Engl) 2014;384(9947):957–979. doi: 10.1016/S0140-6736(14)60497-9. [ DOI ] [ PMC free article ] [ PubMed ] [ Google Scholar ]
- Wasey A., Salen P. StatPearls [Internet] StatPearls Publishing; Treasure Island, FL: 2019. Escherichia coli (E. coli 0157 H7) https://www.ncbi.nlm.nih.gov/books/NBK507845/ (Updated 2019 Feb 3) Available from: [ PubMed ] [ Google Scholar ]
- Weintraub A. Immunology of bacterial polysaccharide antigens. Carbohydr. Res. 2003;338(23):2539–2547. doi: 10.1016/j.carres.2003.07.008. [ DOI ] [ PubMed ] [ Google Scholar ]
- WHO, UNICEF . World Health Organization (WHO) and the United Nations Children’s Fund (UNICEF); Geneva: 2017. Progress on Drinking Water, Sanitation and Hygiene: Update and Sustainable Development Goal Baselines. License: CC BY-NC-SA 3.0 IGO. [ Google Scholar ]
- WHO, UNICEF, World Bank . third ed. 2009. State of the World's Vaccines and Immunization. Geneva. [ Google Scholar ]
- Wiersinga J., Virk S., Torres G., Currie J., Peacock J., Dance A., Limmathurotsakul D. Melioidosis. Nat. Rev. Dis. Prim. 2018;4:17107. doi: 10.1038/nrdp.2017.107. [ DOI ] [ PMC free article ] [ PubMed ] [ Google Scholar ]
- World Health Organization . 1997. Division of Emerging and Communicable Diseases Surveillance and Control Annual Report- 1996. [ Google Scholar ]
- World Health Organization . 2003. Emerging Issues in Water and Infectious Disease. [ Google Scholar ]
- World Health Organization . fourth ed. WHO Press; Geneva, Switzerland: 2011. Guidelines for Drinking-Water Quality. [ Google Scholar ]
- World Health Organization WHO position paper on hepatitis A vaccines-June 2012. Wkly. Epidemiol. Rec. 2012;87(28–29):261–276. [ Google Scholar ]
- World Health Organization . 2019. Dracunculiasis (Guinea-Worm Disease) Fact Sheet. [ Google Scholar ]
- Yu L., Plouffe F., Pastoris C., Stout E., Schousboe M., Widmer A., Summersgill J., File T., Heath M., Paterson L., Chereshsky A. Distribution of Legionella species and serogroups isolated by culture in patients with sporadic community-acquired legionellosis: an international collaborative survey. J. Infect. Dis. 2002;186(1):127–128. doi: 10.1086/341087. [ DOI ] [ PubMed ] [ Google Scholar ]
- View on publisher site
- PDF (841.9 KB)
- Collections
Similar articles
Cited by other articles, links to ncbi databases.
- Download .nbib .nbib
- Format: AMA APA MLA NLM
Add to Collections

IMAGES
COMMENTS
This review focuses on waterborne diseases, its classification and the various methods employed in the bacteriological analysis of water. Diseases related to water and sanitation endemic in Sub...
Common water-borne diseases such as diarrhoea, dysentery, typhoid fever, hepatitis A and cholera are caused by unhealthy water, poor sanitation and poor personal hygiene practices.
Untangling the impacts of climate change on waterborne diseases: a systematic review of relationships between diarrheal diseases and temperature, rainfall, flooding, and drought. Environ Sci ...
The health risks of water-borne diseases, mental health problems, vector-borne diseases, physical health problems, and malnutrition increased during or after floods compared to pre-flood periods or non-flooded areas. To be specific, the effects of a flood on diseases were analyzed for each category.
Waterborne diseases that originated due to pathogen microorganisms are emerging as a serious global health concern. Therefore, rapid, accurate, and specific detection of these microorganisms (i.e., bacteria, viruses, protozoa, and parasitic ...
Waterborne viruses are frequently implicated as the cause of water-related gastrointestinal illness. Waterborne disease outbreaks (WBDOs) are reported each year and are associated with recreational water (RW), treated drinking water (DW), and ground water (treated and untreated).
Contributors to this chapter discuss a broad range of responses to the threat of waterborne disease, including drinking water disinfection, increasing access to water, improving sanitation, and investment in and implementation of public health interventions.
This paper reviews several strategies on their efficacy in combating waterborne diseases, particularly in rural regions of developing countries. Researchers have reported on several different strategies previously.
Heavy rainfall, flooding and hot weather are associated with waterborne diseases, but early warning systems could intercept these cascading risks.
Waterborne pathogens cause diseases in humans through two major exposure pathways: drinking water and recreational waters. This chapter on waterborne pathogens will be starting with an introduction, followed by descriptions on classical waterborne pathogens; bacteria, viruses, protozoans, and helminths placing emphasis on the World Health ...